Razor Pages Exercise
YouTube VideoNow that you know how to create Razor pages, let’s see what makes them useful for creating dynamic web pages.
Initial Project
We’ll start with a simple ASP.NET web app using Razor Pages to display a database of movies. This app consists of a single page, Index
that will be used to display the details of the movies in the database. It also contains classes representing an individual movie (Movie
) and the movie database (MovieDatabase
).
You can clone the starting project from the GitHub Classroom url provided in the Canvas Assignment (for students in the CIS 400 course), or directly from the GitHub repo (for other readers).
Movie Class
Here is the starting point of a class representing a movie:
/// <summary>
/// A class representing a Movie
/// </summary>
public class Movie
{
/// <summary>
/// Gets and sets the title of the movie
/// </summary>
public string Title { get; set; }
/// <summary>
/// Gets and sets the Motion Picture Association of America Rating
/// </summary>
public string MPAARating { get; set; }
/// <summary>
/// Gets and sets the primary genre of the movie
/// </summary>
public string MajorGenre { get; set; }
/// <summary>
/// Gets and sets the Internet Movie Database rating of the movie
/// </summary>
public float? IMDBRating { get; set; }
/// <summary>
/// Gets and sets the Rotten Tomatoes rating of the movie
/// </summary>
public float? RottenTomatoesRating { get; set; }
}
As you can see, it’s a pretty simple data class.
However, we do have one new thing we haven’t seen before - a new use of the question mark symbol (?
) in float?
. This indicates a nullable type, i.e. in addition to the possible float values, the variable can also be null
.
Remember, a float
is a value type, and normally you cannot set a value type to null
. By making it a nullable type, we effectively have wrapped it in an object (technically, a Nullable<T>
object). So instead of RottenTomatoesRating
referencing the memory where the value of the float is stored, it is now storing a reference to that location in memory. This reference can itself be null
if it isn’t pointing anywhere.
We need all of these properties to have the possibility to be null
, as the data we are working with does not have values for all of them. Let’s look at that data next.
Serialized Movie Data
The movies.json file contains data in a JSON format. JSON stands for Javascript Serialization Object Notation. As the name suggests, it is a serialization format - a way of expressing the state of a data object in text. While it originates with JavaScript, JSON has become a popular format for exchanging data in many programming languages. Let’s take a closer look at this file’s structure.
The first movie in the file is The Land Girls:
[
{
"Title": "The Land Girls",
"USGross": 146083,
"WorldWideGross": 146083,
"USDVDSales": null,
"ProductionBudget": 8000000,
"ReleaseDate": "Jun 12 1998",
"MPAARating": "R",
"RunningTime": null,
"Distributor": "Gramercy",
"Source": null,
"MajorGenre": null,
"CreativeType": null,
"Director": null,
"RottenTomatoesRating": null,
"IMDBRating": 6.1,
"IMDBVotes": 1071
},
...
]
The outer square brackets ([
, ]
) indicate that the file contents represent an array. The curly braces ({
, }
) indicate an object - thus the file represents an array of objects. Each object consists of key/value pairs, i.e. "Title": "The Land Girls"
indicates the title of the film. We’re using a library to deserialize these JSON objects into our C# Movie
object structure, so we need the keys to match the property names in that structure.
As you can see with this entry, many of the values are null
. This is why we needed to introduce nullables into our data object - otherwise when we deserialized this object in our C# code, our program would crash when it tried to set one of the value properties to null
.
The MovieDatabase Class
Now let’s look at the MovieDatabase
class:
/// <summary>
/// A class representing a database of movies
/// </summary>
public static class MovieDatabase
{
private static List<Movie> movies = new List<Movie>();
/// <summary>
/// Loads the movie database from the JSON file
/// </summary>
static MovieDatabase() {
using (StreamReader file = System.IO.File.OpenText("movies.json"))
{
string json = file.ReadToEnd();
movies = JsonConvert.DeserializeObject<List<Movie>>(json);
}
}
/// <summary>
/// Gets all movies in the database
/// </summary>
public static IEnumerable<Movie> All { get { return movies; } }
}
}
There are a couple of interesting features. First, the class is static
, which means we cannot construct an instance of it - there will only ever be one instance, and we’ll reference it directly from its class name, MovieDatabase
. As a static class, all of its fields, methods, and properties must likewise be declared static
. If these are public, then we can access them directly from the class name, i.e. to access the All
property, we would invoke MovieDatabase.All
.
Notice that we have declared a static constructor for the class. This will be invoked the first time the class is used in the program, and only that one time (as after that, the one static instance of the class exists). Since we cannot construct a static class directly, there is no reason to add an access modifier (public
, protected
, or private
).
The usual reason to have a static constructor is to do some kind of initialization, and that is what we are doing here. We are loading the JSON file, and using the JsonConvert.DeserializeObject<T>()
method to convert the JSON into a List<Movie>
. This method is part of the JSON.net library from Newtonsoft - which is provided to us through a Nuget package. If you look under the Dependencies entry in the solution explorer, you can find a Packages list that contains Newtonsoft.JSON, this library.
Nuget is a package manager that allows developers to publish .NET libraries they have created for other developers to use. It is a source of many useful libraries, and if you become a professional .NET developer, it is probably a resource you will find yourself using often.
Displaying a list of Movie Titles
Okay, now that we’re familiar with the starter code, let’s turn our attention to the task at hand - we’d like to display all the movies in our database on the Index
razor page.
Refactoring Index.cshtml
Let’s start by looking at our Page class, Index.cshtml
:
@page
@model IndexModel
@{
ViewData["Title"] = "Home page";
}
<h1>Hello World</h1>
Let’s change the header Hello World
to say Movies
. And below that, let’s declare an unordered list of movie titles, and put the first few titles in list items:
<h1>Movies</h1>
<ul>
<li>The Land Girls</li>
<li>First Love, Last Rites</li>
<li>I Married a Strange Person</li>
</ul>
Go ahead and run your program. Your page should look like:
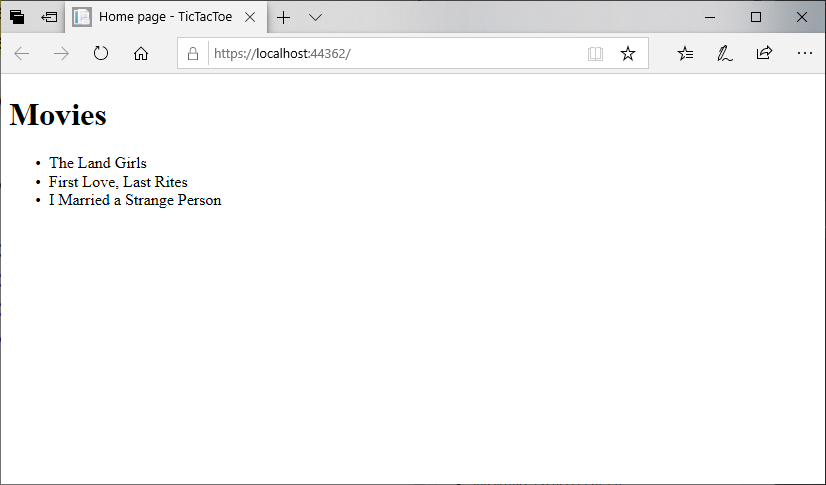
This approach would work fine, but there are 3,201 entries in our database - do you really want to do that by hand?
Instead, let’s leverage the power of Razor templates, and use some C# code to iterate through each entry in the database. We can do this with a foreach
loop, just like you might do in a regular C# class:
<h1>Movies</h1>
<ul>
@foreach(Movie movie in MovieDatabase.All)
{
<li>@movie.Title</li>
}
</ul>
Notice that inside the body of the foreach
loop, we use regular HTML to declare a list item element (<li>
). But for the content of that element, we are using the movie.Title
property. As this is prefaced with an at symbol (@
), the Razor template engine evaluates it as a C# expression, and concatenates the result (the movie’s title) into the list item. Thus, for the first item in the database, <li>The Land Girls</li>
.
Each of these is in turn concatenated into the page as the foreach
loop is processed, resulting in a list of all the movie titles in the database. Run the program and see for yourself:
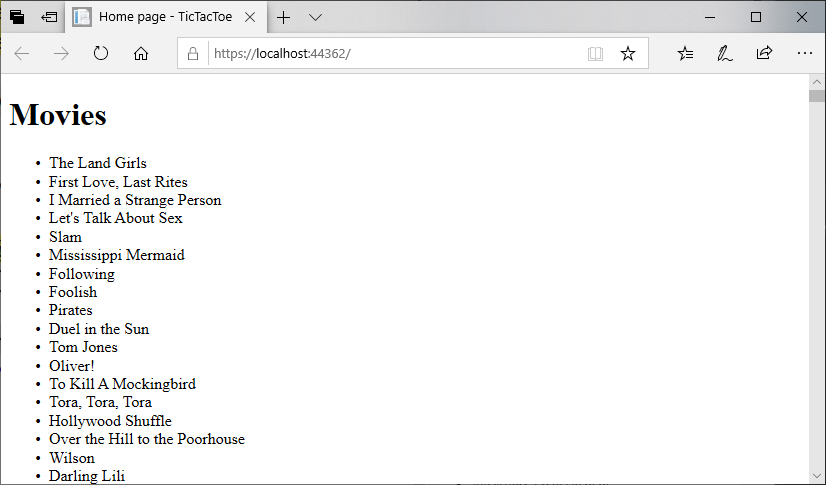
They’re all there. You can scroll all the way to the bottom.
Adding Some Detail
It might be interesting to see more information about the movies than just the title. Let’s take advantage of the details in our Movie
class by expanding what is shown:
<h1>Movies</h1>
<ul>
@foreach(Movie movie in MovieDatabase.All)
{
<li>
<h3>@movie.Title</h3>
<div>@movie.MPAARating</div>
<div>@movie.MajorGenre</div>
</li>
}
</ul>
Notice that unlike our WPF XAML, we can nest as many children in an HTML element as we want! If we run the program now:
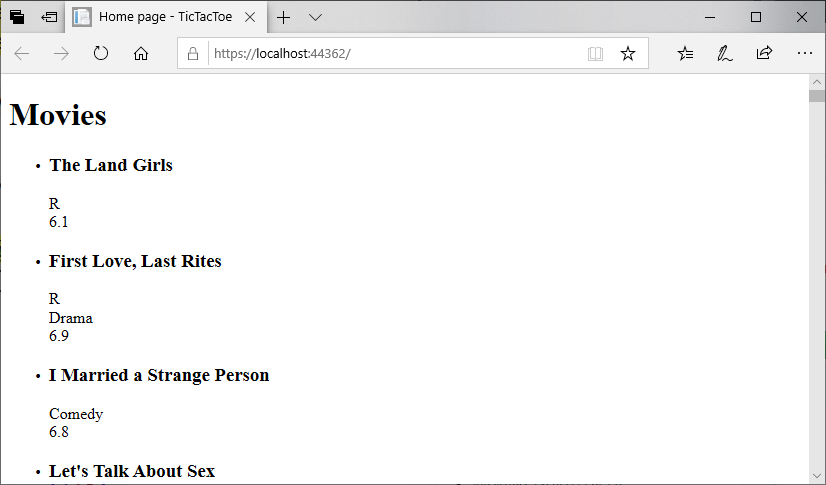
Well, it works, but it’s also underwhelming (and a bit difficult to interpret). Notice that our first few movies don’t have all the rating properties, so there are large blank spaces.
Let’s take advantage of Razor’s ability to use conditionals to leave those blanks out:
<h1>Movies</h1>
<ul class="movie-list">
@foreach(Movie movie in MovieDatabase.All)
{
<li>
<h3 class="title">@movie.Title</h3>
@if (movie.MPAARating != null)
{
<div class="mpaa">
Rated @movie.MPAARating
</div>
}
@if (movie.MajorGenre != null)
{
<div class="genre">
@movie.MajorGenre
</div>
}
</li>
}
</ul>
We’ve also added the text “Rated” before our MPAARating
, so the entry will now read “Rated R” for an R-rated movie, “Rated G” for a g-rated movie, and so on.
We also added class attributes to the <h3>
and each <div>
, as well as the movie list itself. We’ll use these to style our elements.
Adding Some Style
We can find our CSS rules for the project in wwwroot/css/site.js.
Let’s start with the unordered list itself. We can select it with the ul.movie-list
selector. We’ll remove any padding and margins, and add a solid line above it:
ul.movie-list {
padding: 0;
margin: 0;
border-top: 1px solid gray;
}
We’ll then select each list item that is a child of that list with ul.movie-list > li
. We’ll remove the bullet, add a lighter border at the bottom to separate our items, and put a 10-pixel margin all the way around:
ul.movie-list > li {
list-style-type: none;
border-bottom: 1px solid lightgray;
margin: 10px;
}
You might wonder why we put the list in an unordered list at all, if we’re just going to change all its default styles. Remember, HTML provides the structure as well as the content. By putting the items in a list, we’re signifying that the items are a list. We are conveying semantic meaning with the structure we use.
Remember, it’s not just humans that read the internet. Many bots and algorithms do as well, and they typically won’t use the lens of CSS styling - they’ll be reading the raw HTML.
We’ll make our title headers a dark slate gray, have a slightly larger-then-normal text, and remove the margins so that there are no large space between the header and the text is directly above and beneath them:
.title {
color: darkslategray;
font-size: 1.2rem;
margin: 0;
}
Finally, let’s lighten the color of the MPAA rating and genre:
.mpaa {
color: slategray;
}
.genre {
color: lightslategray;
}
Adding Some Ratings
While the MPAA ratings convey the age-appropriateness of a movie, the IMDB and Rotten Tomatoes ratings provide a sense of how much people enjoy the films. Since this probably information our readers might want to see to help them judge what films to look at, it might be nice to call attention to them in some way.
What if we put them into their own boxes, and position them on the right side of the screen, opposite the title? Something like:
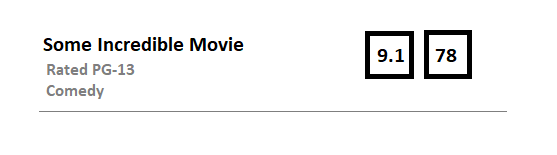
There are many ways of accomplishing this look, including the float
property or using a <table>
element. But let’s turn to one of the newer and more powerful css layout features, the flexbox layout.
We’ll start by refactoring our HTML slightly, to divide our <li>
into two <div>
s, one containing our current details for the movie, and one for the viewer ratings:
<li>
<div class="details">
<h3>@movie.Title</h3>
@if (movie.MPAARating != null)
{
<div class="mpaa">
Rated @movie.MPAARating
</div>
}
@if (movie.MajorGenre != null)
{
<div class="genre">
@movie.MajorGenre
</div>
}
</div>
<div class="ratings">
@if (movie.IMDBRating != null)
{
<div class="imdb">
@movie.IMDBRating
</div>
}
@if (movie.RottenTomatoesRating != null)
{
<div class="rotten-tomatoes">
@movie.RottenTomatoesRating
</div>
}
</div>
</li>
Now we’ll apply the flexbox properties and a minimum height to the list item:
ul.movie-list > li {
display: flex;
flex-direction: row;
align-items: flex-start;
justify-content: space-between;
min-height: 50px;
}
These can be combined with our earlier rule block with the same selector, or they can be declared separately.
We’ll also use flexbox to make our ratings appear side-by-side:
.ratings {
display: flex;
flex-direction: row;
}
And use some styles to add the border, center the text, and use gray for the text and border colors:
.imdb, .rotten-tomatoes {
color: gray;
border: 1px solid gray;
width: 60px;
text-align: center;
font-size: 1.2rem;
}
Notice that we can use the comma to allow more than one selector to share a rule.
It might be nice to label the two ratings, as Rotten Tomatoes is on a 100-point scale, and IMDB uses a 10-point scale. We could go back and apply this in the HTML, but it is a good opportunity to show off the ::before
pseduo-selector, which allows us to create HTML elements using css:
.imdb::before {
content: "IMDB";
display: block;
font-size: 1rem;
}
.rotten-tomatoes::before {
content: "Rotten";
display: block;
font-size: 1rem;
}
If you run your code at this point, you may notice your <h3>
styles have stopped applying. If we look at the selector, we’ll see why. It is currently: ul.movie-list > li > h3
, which indicates the <h3>
it applies to should be a direct child of the <li>
tag. We could swap to using h3
instead, but this would apply to all <h3>
tags on our page. Instead, let’s swap the >
for a space
, which indicates a descendant instead of a direct child. In fact, we could drop the li
as well:
ul.movie-list h3 {
font-size: 1.2rem;
margin-bottom: 0;
color: darkslategray;
}
The end result is very close to our sketch:
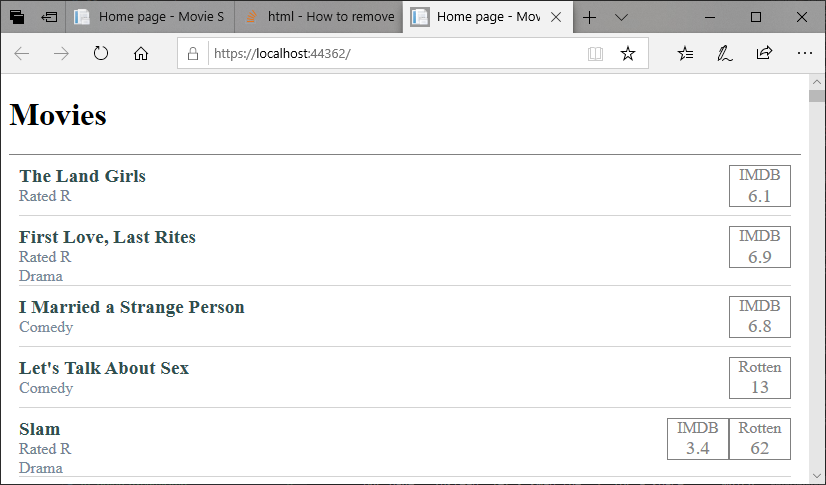
Clearly, CSS is a powerful tool. It can be challenging to learn, but if you are going to be involved in web development, it is time well spent.
The MDN CSS documentation and the CSS-Tricks site are both excellent references for learning CSS.
Subsections of Web Data
Adding Search to the Movie Site
Let’s add a search form and functionality to our movie website. We’ll add this to our Index.cshtml page, just above the <h1>
element:
<form>
<input type="text" name="SearchTerms"/>
<input type="submit" value="Search">
</form>
<h1>Movie Results</h1>
We’ll also change the <h1>
contents to “Movie Results”.
Try typing a search term into the search box, and click the search button. Has anything changed?
The Request Object
When you click the search button, the browser serializes your form, and makes a request against your server including the search terms. By default this request is a GET request, and the contents of the form are serialized using urlencoding (aka percent encoding), a special string format. This string is then appended to the requested url as the query string (aka search string) - a series of key-value pairs proceeded by the question mark symbol(?
) and separated by the ampersand (&
).
This data is made available to us in our PageModel Index.cshtml.cs by ASP.NET. Let’s take a look at it now. Notice the method public void OnGet()
? This method is invoked every time the page is requested using a GET request. Thus, if we need to do some initialization and/or processing, this would be the place to do it.
Inside the PageModel, we can access the request data using the Request
object. The exact string can be accessed with Request.QueryString
, or the parsed and deserialized results can be accessed from Request.Query
. Let’s use the latter to pull out the search terms:
public void OnGet()
{
String terms = Request.Query["SearchTerms"];
}
We can store that value, and make it available to the page itself, by creating a public property. Let’s create one named SearchTerms
:
public string SearchTerms { get; set; }
And we’ll refactor our OnGet()
to store the search terms coming in from the request:
public void OnGet()
{
SearchTerms = Request.Query["SearchTerms"];
}
Now we can refactor our input element to use that public property from our model as its default value:
<input type="text" name="SearchTerms" value="@Model.SearchTerms"/>
The first time we visit the index page, the SearchTerms
value will be null, so our input would have value=""
. The browser interprets this as empty. If we add a search term and click the search button, we’ll see the page reload. And since @Model.SearchTerms
has a value this time, we’ll see that string appear in search box!
Now we just need to search for those terms…
Adding Search to the Database
We’ll start by defining a new static method in our MovieDatabase.cs file to search for movies using the search terms:
/// <summary>
/// Searches the database for matching movies
/// </summary>
/// <param name="terms">The terms to search for</param>
/// <returns>A collection of movies</returns>
public static IEnumerable<Movie> Search(string terms)
{
// TODO: Search database
}
We’ll need a collection of results that implements the IEnumerable<T>
interface. Let’s use the familiar List<T>
:
List<Movie> results = new List<Movie>();
Now, there is a chance that the search terms we receive are null
. If that’s the case, we would either 1) return all the movies, or 2) return no movies. You can choose either option, but for now, I’ll return all movies
// Return all movies if there are no search terms
if(terms == null) return All;
If we do have search terms, we need to add any movies from our database that include those terms in the title. This requires us to check each movie in our database:
// return each movie in the database containing the terms substring
foreach(Movie movie in All)
{
if(movie.Title.Contains(terms, StringComparison.InvariantCultureIgnoreCase))
{
results.Add(movie);
}
}
We’ll use String.Contains() to determine if our terms are a substring within the title, ignoring case differences. If we find it, we’ll add the movie to our results
list.
Finally, we’ll return that list:
Now, we can refactor our Index.cshtml.cs to use this new search method:
/// <summary>
/// The movies to display on the index page
/// </summary>
public IEnumerable<Movie> Movies { get; protected set; }
/// <summary>
/// The current search terms
/// </summary>
public string SearchTerms { get; set; }
/// <summary>
/// Gets the search results for display on the page
/// </summary>
public void OnGet()
{
SearchTerms = Request.Query["SearchTerms"];
Movies = MovieDatabase.Search(SearchTerms);
}
We’ll also need ot refactor our Index.cshtml.cs to use the search results, instead of the entire database:
<ul class="movie-list">
@foreach(Movie movie in @Model.Movies)
{
<li>
<div class="details">
<h3 class="title">@movie.Title</h3>
<div class="mpaa">@movie.MPAARating</div>
<div class="genre">@movie.MajorGenre</div>
</div>
<div class="ratings">
@if(movie.IMDBRating != null)
{
<div class="imdb">
@movie.IMDBRating
</div>
}
@if(movie.RottenTomatoesRating != null)
{
<div class="rotten-tomatoes">
@movie.RottenTomatoesRating
</div>
}
</div>
</li>
}
</ul>
If we try running the project again, and searching for the term “Love”… it crashes? What is going on?
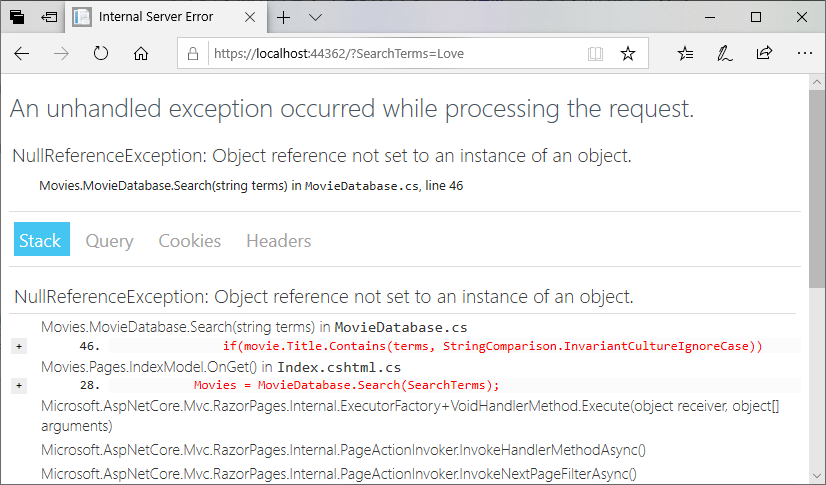
Notice that the error is a NullReferenceException
, and occurs in our if
statement checking the title.
Bad Data
If we think about what variables are involved in the line if(movie.Title.Contains(terms, StringComparison.InvariantCultureIgnoreCase))
, we have:
Which of these three values can be null? We know for certain terms
is not, as we test for the null value and return if it exists just before this portion of our code. Similarly, movie
cannot be null, as it is an entry in the list provided by All
, and if it were null, our page would have crashed before we added searching. That leaves movie.Title
as a possibility.
If we comb through the data in movies.json, we find on line 54957 a movie with null
for a title:
{
"Title": null,
"USGross": 26403,
"WorldwideGross": 3080493,
"USDVDSales": null,
"ProductionBudget": 3700000,
"ReleaseDate": "Nov 03 2006",
"MPAARating": "Not Rated",
"RunningTime": 85,
"Distributor": "IFC Films",
"Source": "Original Screenplay",
"MajorGenre": "Thriller/Suspense",
"CreativeType": "Contemporary Fiction",
"Director": null,
"RottenTomatoesRating": 39,
"IMDBRating": 6.6,
"IMDBVotes": 11986
},
Working from the provided metadata, we can eventually identify the film as one titled Unknown. It would seem that whomever wrote the script to create this JSON file interpreted “Unknown” to mean the title was unknown (hence null), rather than the literal word “Unknown”.
If we dig deeper into the JSON file, we can find other issues. For example, the JSON identifies the controversial film Birth of a Nation as being released in 2015, when it was actually the first full-length theatrical film ever released, in 1915! Most likely the original database from which these entries were derived only used two digits for the year, i.e. 15
, and the scripter who converted it to JSON chose a threshold date to determine if it was released in the 20 or 21st century, i.e.:
if(date < 28)
{
date += 2000;
}
else {
date += 1900;
}
The earliest movie release date in the JSON is 1928, for “The Broadway Melody”, which suggests that all the movies released between 1915 and 1928 have been mislabeled as being released in the 21st century!
Unfortunately, these kinds of errors are rampant in databases, so as software developers we must be aware that our data may well be dirty - containing erroneous values, and anticipate these errors much like we do with user input. It is a good idea to clean up and fix these errors in our database so that it will be more reliable, but we also need to check for potential errors in our own code, as the database could be updated with more junk data in the future. Thus, we’ll add a null
check to our if
statement in MovieDatabase.cs:
if(movie.Title != null && movie.Title.Contains(terms, StringComparison.InvariantCultureIgnoreCase))
This will protect us against a NullReferenceException
when our movie titles are null. Now if you try the search again, you should see the results:
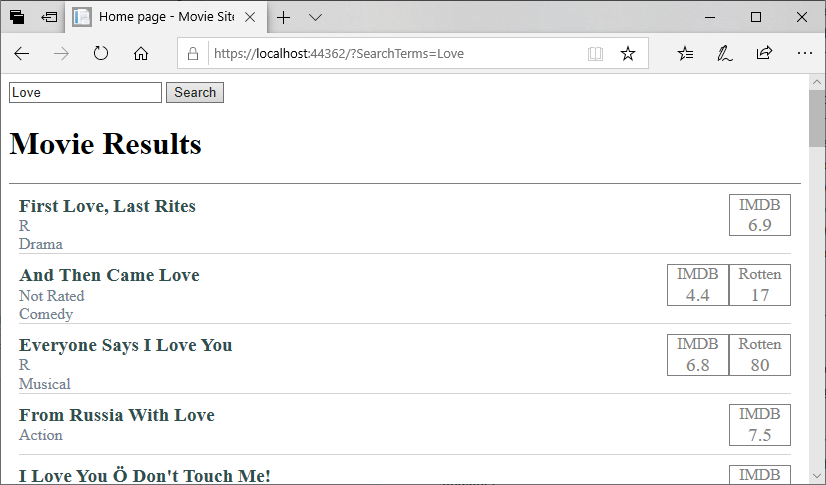
Adding Categorical Filters to the Movie Site
Let’s add some filters to the page as well. We’ll start with categorical filters, i.e. filtering by category. Let’s start by filtering for MPAA Rating. We know that there are only a handful of ratings issued by the Motion Picture Association of America - G, PG, PG-13, R, and NC-17. We might be tempted to use an enumeration to represent these values, but in C# an enumeration cannot have strings for values. Nor can we use the hyphen (-
) in an enumeration name.
Defining the MPAA Ratings
So let’s define a string array with our MPAA values, and make it accessible from our MovieDatabase
class:
/// <summary>
/// Gets the possible MPAARatings
/// </summary>
public static string[] MPAARatings
{
get => new string[]
{
"G",
"PG",
"PG-13",
"R",
"NC-17"
};
}
Now in our <form>
in Index.cshtml we can add a checkbox for each of these possible values:
<form>
<input type="text" name="SearchTerms" value="@Model.SearchTerms"/>
<input type="submit" value="Search">
@foreach (string rating in MovieDatabase.MPAARating)
{
<label>
<input type="checkbox" name="MPAARatings" value="@rating"/>
@rating
</label>
}
</form>
If you try running the project now, and check a few boxes, you’ll see the query string results look something like:
?SearchTerms=&MPAARatings=G&MPAARatings=PG-13
Notice how the key MPAARatings is repeated twice? What would that look like in our PageModel? We can find out; declare a var
to hold the value in the OnGet()
method of Index.cshtml.cs:
var MPAARatings = Request.Query["MPAARatings"];
If we add a breakpoint on this line, and run our code, then check several boxes (you’ll have to continue the first time you hit the breakpoint), then step over the line, we’ll see that the var MPAA
rating is set to a string collection. We could therefore store it in an array property in Index.cshtml.cs, much like we did with our SearchTerms
:
/// <summary>
/// The filtered MPAA Ratings
/// </summary>
public string[] MPAARatings { get; set; }
And we can refactor the line we just added to OnGet()
to use this new property:
MPAARatings = Request.Query["MPAARatings"];
Then, in our Index.cshtml Razor Page, we can refactor the checkbox to be checked if we filtered against this rating in our last request:
<input type="checkbox" name="MPAARatings" value="@rating" checked="@Model.MPAARatings.Contains(rating)"/>
Now our filters stick around when we submit the search request. That just leaves making the filters actually work.
Applying MPAA Rating Filters
Let’s add another method to our MovieDatabase
class, FilterByMPAARating()
:
/// <summary>
/// Filters the provided collection of movies
/// </summary>
/// <param name="movies">The collection of movies to filter</param>
/// <param name="ratings">The ratings to include</param>
/// <returns>A collection containing only movies that match the filter</returns>
public static IEnumerable<Movie> FilterByMPAARating(IEnumerable<Movie> movies, IEnumerable<string> ratings)
{
// TODO: Filter the list
}
Notice that in this method, we accept an IEnumerable<Movie>
parameter. This is the list of movies we want to filter. We use this, instead of the All()
we did in the Search()
method, as we would want to filter the results of a search.
Let’s do a null/empty check, and just return this shortlist if no filters are specified:
// If no filter is specified, just return the provided collection
if (ratings == null || ratings.Count() == 0) return movies;
Otherwise, we’ll use the same process we did before. Start with an empty list of movies, and iterate over the collection seeing if any match. However, as we have two collections (the movies and the ratings), we’ll see if the ratings collection contains the supplied movie’s rating.
// Filter the supplied collection of movies
List<Movie> results = new List<Movie>();
foreach(Movie movie in movies)
{
if(movie.MPAARating != null && ratings.Contains(movie.MPAARating))
{
results.Add(movie);
}
}
Finally, we’ll return our results:
Now, back in our PageModel Index.cshtml.cs, we’ll apply our filter to the results of our search. The refactored OnGet()
should then be:
public void OnGet()
{
SearchTerms = Request.Query["SearchTerms"];
MPAARatings = Request.Query["MPAARatings"];
Movies = MovieDatabase.Search(SearchTerms);
Movies = MovieDatabase.FilterByMPAARating(Movies, MPAARatings);
}
Now we can run a search with filters applied. For example, searching for the word “Love” and movies that are PG or PG-13 yields:
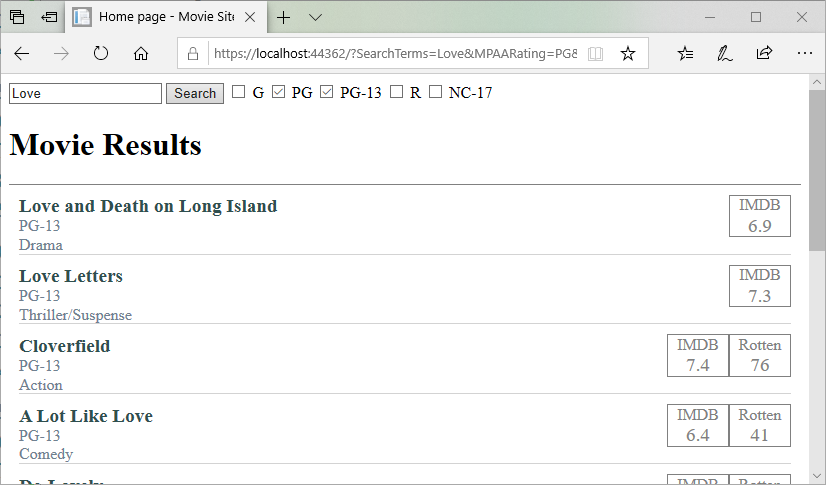
You might be wondering why Cloverfield is listed. But remember, we’re searching by substring, and C LOVE rfield contains love!
Filtering by Genre
Let’s add filters for genre next. But what genres should be included? This is not as clear-cut as our MPAA rating, as there is no standards organization that says “these are the only offical genres that exist.” In fact, new genres emerge from time to time. So a better source of this info might just be to see what Genres are defined in our data, i.e.:
HashSet<string> genres = new HashSet<string>();
foreach(Movie movie in All) {
if(movie.MajorGenre != null)
{
genres.Add(movie.MajorGenre);
}
}
Here we use a HashSet instead of a list, as it only adds each unique item once. Duplicates are ignored.
But where would this code go? We could place it in a getter for MovieDatabase.Genres
:
public IEnumerable<string> Genres
{
get
{
HashSet<string> genres = new HashSet<string>();
foreach(Movie movie in All) {
if(movie.MajorGenre != null)
{
genres.Add(movie.MajorGenre);
}
}
}
}
But this means that every time we want to access it, we’ll search through all the movies… This is an O(n) operation, and will make our website slower.
Instead, let’s create a private static variable in the MovieDatabase
class to cache this collection as an array of strings:
// The genres represented in the database
private static string[] _genres;
And expose it with a public static property:
/// <summary>
/// Gets the movie genres represented in the database
/// </summary>
public static string[] Genres => _genres;
And finally, we’ll populate this array in the static constructor of MovieDatabase
, after the JSON file has been processed:
HashSet<string> genreSet = new HashSet<string>();
foreach(Movie movie in _movies) {
if(movie.MajorGenre != null)
{
genreSet.Add(movie.MajorGenre);
}
}
_genres = genreSet.ToArray();
This approach means the finding of genres only happens once, and getting the Genre
property is a constant-time O(1) operation.
We can implement the filters for the genres in the same way as we did for the MPAA filters; I’ll leave that as an exercise for the reader.
Adding Categorical Filters to the Movie Site
Let’s add some filters to the page as well. We’ll start with categorical filters, i.e. filtering by category. Let’s start by filtering for MPAA Rating. We know that there are only a handful of ratings issued by the Motion Picture Association of America - G, PG, PG-13, R, and NC-17. We might be tempted to use an enumeration to represent these values, but in C# an enumeration cannot have strings for values. Nor can we use the hyphen (-
) in an enumeration name.
Defining the MPAA Ratings
So let’s define a string array with our MPAA values, and make it accessible from our MovieDatabase
class:
/// <summary>
/// Gets the possible MPAARatings
/// </summary>
public static string[] MPAARatings
{
get => new string[]
{
"G",
"PG",
"PG-13",
"R",
"NC-17"
};
}
Now in our <form>
in Index.cshtml we can add a checkbox for each of these possible values:
<form>
<input type="text" name="SearchTerms" value="@Model.SearchTerms"/>
<input type="submit" value="Search">
@foreach (string rating in MovieDatabase.MPAARating)
{
<label>
<input type="checkbox" name="MPAARatings" value="@rating"/>
@rating
</label>
}
</form>
If you try running the project now, and check a few boxes, you’ll see the query string results look something like:
?SearchTerms=&MPAARatings=G&MPAARatings=PG-13
Notice how the key MPAARatings is repeated twice? What would that look like in our PageModel? We can find out; declare a var
to hold the value in the OnGet()
method of Index.cshtml.cs:
var MPAARatings = Request.Query["MPAARatings"];
If we add a breakpoint on this line, and run our code, then check several boxes (you’ll have to continue the first time you hit the breakpoint), then step over the line, we’ll see that the var MPAA
rating is set to a string collection. We could therefore store it in an array property in Index.cshtml.cs, much like we did with our SearchTerms
:
/// <summary>
/// The filtered MPAA Ratings
/// </summary>
public string[] MPAARatings { get; set; }
And we can refactor the line we just added to OnGet()
to use this new property:
MPAARatings = Request.Query["MPAARatings"];
Then, in our Index.cshtml Razor Page, we can refactor the checkbox to be checked if we filtered against this rating in our last request:
<input type="checkbox" name="MPAARatings" value="@rating" checked="@Model.MPAARatings.Contains(rating)"/>
Now our filters stick around when we submit the search request. That just leaves making the filters actually work.
Applying MPAA Rating Filters
Let’s add another method to our MovieDatabase
class, FilterByMPAARating()
:
/// <summary>
/// Filters the provided collection of movies
/// </summary>
/// <param name="movies">The collection of movies to filter</param>
/// <param name="ratings">The ratings to include</param>
/// <returns>A collection containing only movies that match the filter</returns>
public static IEnumerable<Movie> FilterByMPAARating(IEnumerable<Movie> movies, IEnumerable<string> ratings)
{
// TODO: Filter the list
}
Notice that in this method, we accept an IEnumerable<Movie>
parameter. This is the list of movies we want to filter. We use this, instead of the All()
we did in the Search()
method, as we would want to filter the results of a search.
Let’s do a null/empty check, and just return this shortlist if no filters are specified:
// If no filter is specified, just return the provided collection
if (ratings == null || ratings.Count() == 0) return movies;
Otherwise, we’ll use the same process we did before. Start with an empty list of movies, and iterate over the collection seeing if any match. However, as we have two collections (the movies and the ratings), we’ll see if the ratings collection contains the supplied movie’s rating.
// Filter the supplied collection of movies
List<Movie> results = new List<Movie>();
foreach(Movie movie in movies)
{
if(movie.MPAARating != null && ratings.Contains(movie.MPAARating))
{
results.Add(movie);
}
}
Finally, we’ll return our results:
Now, back in our PageModel Index.cshtml.cs, we’ll apply our filter to the results of our search. The refactored OnGet()
should then be:
public void OnGet()
{
SearchTerms = Request.Query["SearchTerms"];
MPAARatings = Request.Query["MPAARatings"];
Movies = MovieDatabase.Search(SearchTerms);
Movies = MovieDatabase.FilterByMPAARating(Movies, MPAARatings);
}
Now we can run a search with filters applied. For example, searching for the word “Love” and movies that are PG or PG-13 yields:
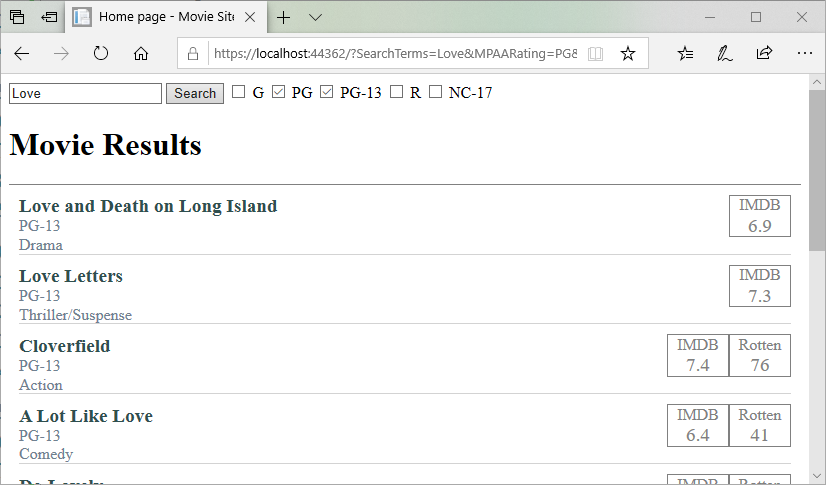
You might be wondering why Cloverfield is listed. But remember, we’re searching by substring, and C LOVE rfield contains love!
Filtering by Genre
Let’s add filters for genre next. But what genres should be included? This is not as clear-cut as our MPAA rating, as there is no standards organization that says “these are the only offical genres that exist.” In fact, new genres emerge from time to time. So a better source of this info might just be to see what Genres are defined in our data, i.e.:
HashSet<string> genres = new HashSet<string>();
foreach(Movie movie in All) {
if(movie.MajorGenre != null)
{
genres.Add(movie.MajorGenre);
}
}
Here we use a HashSet instead of a list, as it only adds each unique item once. Duplicates are ignored.
But where would this code go? We could place it in a getter for MovieDatabase.Genres
:
public IEnumerable<string> Genres
{
get
{
HashSet<string> genres = new HashSet<string>();
foreach(Movie movie in All) {
if(movie.MajorGenre != null)
{
genres.Add(movie.MajorGenre);
}
}
}
}
But this means that every time we want to access it, we’ll search through all the movies… This is an O(n) operation, and will make our website slower.
Instead, let’s create a private static variable in the MovieDatabase
class to cache this collection as an array of strings:
// The genres represented in the database
private static string[] _genres;
And expose it with a public static property:
/// <summary>
/// Gets the movie genres represented in the database
/// </summary>
public static string[] Genres => _genres;
And finally, we’ll populate this array in the static constructor of MovieDatabase
, after the JSON file has been processed:
HashSet<string> genreSet = new HashSet<string>();
foreach(Movie movie in _movies) {
if(movie.MajorGenre != null)
{
genreSet.Add(movie.MajorGenre);
}
}
_genres = genreSet.ToArray();
This approach means the finding of genres only happens once, and getting the Genre
property is a constant-time O(1) operation.
We can implement the filters for the genres in the same way as we did for the MPAA filters; I’ll leave that as an exercise for the reader.
Adding Categorical Filters to the Movie Site
Let’s add some filters to the page as well. We’ll start with categorical filters, i.e. filtering by category. Let’s start by filtering for MPAA Rating. We know that there are only a handful of ratings issued by the Motion Picture Association of America - G, PG, PG-13, R, and NC-17. We might be tempted to use an enumeration to represent these values, but in C# an enumeration cannot have strings for values. Nor can we use the hyphen (-
) in an enumeration name.
Defining the MPAA Ratings
So let’s define a string array with our MPAA values, and make it accessible from our MovieDatabase
class:
/// <summary>
/// Gets the possible MPAARatings
/// </summary>
public static string[] MPAARatings
{
get => new string[]
{
"G",
"PG",
"PG-13",
"R",
"NC-17"
};
}
Now in our <form>
in Index.cshtml we can add a checkbox for each of these possible values:
<form>
<input type="text" name="SearchTerms" value="@Model.SearchTerms"/>
<input type="submit" value="Search">
@foreach (string rating in MovieDatabase.MPAARatings)
{
<label>
<input type="checkbox" name="MPAARatings" value="@rating"/>
@rating
</label>
}
</form>
If you try running the project now, and check a few boxes, you’ll see the query string results look something like:
?SearchTerms=&MPAARatings=G&MPAARatings=PG-13
Notice how the key MPAARatings is repeated twice? What would that look like in our PageModel? We can find out; declare a var
to hold the value in the OnGet()
method of Index.cshtml.cs:
var MPAARatings = Request.Query["MPAARatings"];
If we add a breakpoint on this line, and run our code, then check several boxes (you’ll have to continue the first time you hit the breakpoint), then step over the line, we’ll see that the var MPAA
rating is set to a string collection. We could therefore store it in an array property in Index.cshtml.cs, much like we did with our SearchTerms
:
/// <summary>
/// The filtered MPAA Ratings
/// </summary>
public string[] MPAARatings { get; set; }
And we can refactor the line we just added to OnGet()
to use this new property:
MPAARatings = Request.Query["MPAARatings"];
Then, in our Index.cshtml Razor Page, we can refactor the checkbox to be checked if we filtered against this rating in our last request:
<input type="checkbox" name="MPAARatings" value="@rating" checked="@Model.MPAARatings.Contains(rating)"/>
Now our filters stick around when we submit the search request. That just leaves making the filters actually work.
Applying MPAA Rating Filters
Let’s add another method to our MovieDatabase
class, FilterByMPAARating()
:
/// <summary>
/// Filters the provided collection of movies
/// </summary>
/// <param name="movies">The collection of movies to filter</param>
/// <param name="ratings">The ratings to include</param>
/// <returns>A collection containing only movies that match the filter</returns>
public static IEnumerable<Movie> FilterByMPAARating(IEnumerable<Movie> movies, IEnumerable<string> ratings)
{
// TODO: Filter the list
}
Notice that in this method, we accept an IEnumerable<Movie>
parameter. This is the list of movies we want to filter. We use this, instead of the All()
we did in the Search()
method, as we would want to filter the results of a search.
Let’s do a null/empty check, and just return this shortlist if no filters are specified:
// If no filter is specified, just return the provided collection
if (ratings == null || ratings.Count() == 0) return movies;
Otherwise, we’ll use the same process we did before. Start with an empty list of movies, and iterate over the collection seeing if any match. However, as we have two collections (the movies and the ratings), we’ll see if the ratings collection contains the supplied movie’s rating.
// Filter the supplied collection of movies
List<Movie> results = new List<Movie>();
foreach(Movie movie in movies)
{
if(movie.MPAARating != null && ratings.Contains(movie.MPAARating))
{
results.Add(movie);
}
}
Finally, we’ll return our results:
Now, back in our PageModel Index.cshtml.cs, we’ll apply our filter to the results of our search. The refactored OnGet()
should then be:
public void OnGet()
{
SearchTerms = Request.Query["SearchTerms"];
MPAARatings = Request.Query["MPAARatings"];
Movies = MovieDatabase.Search(SearchTerms);
Movies = MovieDatabase.FilterByMPAARating(Movies, MPAARatings);
}
Now we can run a search with filters applied. For example, searching for the word “Love” and movies that are PG or PG-13 yields:
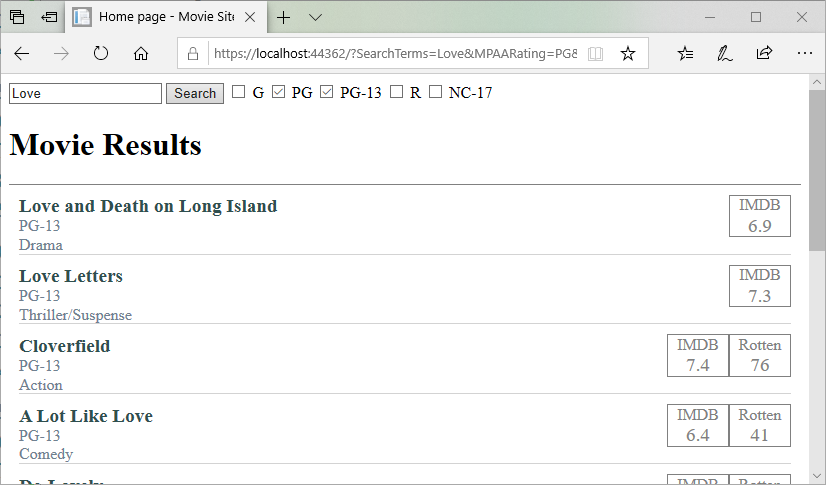
You might be wondering why Cloverfield is listed. But remember, we’re searching by substring, and C LOVE rfield contains love!
Filtering by Genre
Let’s add filters for genre next. But what genres should be included? This is not as clear-cut as our MPAA rating, as there is no standards organization that says “these are the only offical genres that exist.” In fact, new genres emerge from time to time. So a better source of this info might just be to see what Genres are defined in our data, i.e.:
HashSet<string> genres = new HashSet<string>();
foreach(Movie movie in All) {
if(movie.MajorGenre != null)
{
genres.Add(movie.MajorGenre);
}
}
Here we use a HashSet instead of a list, as it only adds each unique item once. Duplicates are ignored.
But where would this code go? We could place it in a getter for MovieDatabase.Genres
:
public IEnumerable<string> Genres
{
get
{
HashSet<string> genres = new HashSet<string>();
foreach(Movie movie in All) {
if(movie.MajorGenre != null)
{
genres.Add(movie.MajorGenre);
}
}
}
}
But this means that every time we want to access it, we’ll search through all the movies… This is an O(n) operation, and will make our website slower.
Instead, let’s create a private static variable in the MovieDatabase
class to cache this collection as an array of strings:
// The genres represented in the database
private static string[] _genres;
And expose it with a public static property:
/// <summary>
/// Gets the movie genres represented in the database
/// </summary>
public static string[] Genres => _genres;
And finally, we’ll populate this array in the static constructor of MovieDatabase
, after the JSON file has been processed:
HashSet<string> genreSet = new HashSet<string>();
foreach(Movie movie in _movies) {
if(movie.MajorGenre != null)
{
genreSet.Add(movie.MajorGenre);
}
}
_genres = genreSet.ToArray();
This approach means the finding of genres only happens once, and getting the Genre
property is a constant-time O(1) operation.
We can implement the filters for the genres in the same way as we did for the MPAA filters; I’ll leave that as an exercise for the reader.
Adding Categorical Filters to the Movie Site
Let’s add some filters to the page as well. We’ll start with categorical filters, i.e. filtering by category. Let’s start by filtering for MPAA Rating. We know that there are only a handful of ratings issued by the Motion Picture Association of America - G, PG, PG-13, R, and NC-17. We might be tempted to use an enumeration to represent these values, but in C# an enumeration cannot have strings for values. Nor can we use the hyphen (-
) in an enumeration name.
Defining the MPAA Ratings
So let’s define a string array with our MPAA values, and make it accessible from our MovieDatabase
class:
/// <summary>
/// Gets the possible MPAARatings
/// </summary>
public static string[] MPAARatings
{
get => new string[]
{
"G",
"PG",
"PG-13",
"R",
"NC-17"
};
}
Now in our <form>
in Index.cshtml we can add a checkbox for each of these possible values:
<form>
<input type="text" name="SearchTerms" value="@Model.SearchTerms"/>
<input type="submit" value="Search">
@foreach (string rating in MovieDatabase.MPAARatings)
{
<label>
<input type="checkbox" name="MPAARatings" value="@rating"/>
@rating
</label>
}
</form>
If you try running the project now, and check a few boxes, you’ll see the query string results look something like:
?SearchTerms=&MPAARatings=G&MPAARatings=PG-13
Notice how the key MPAARatings is repeated twice? What would that look like in our PageModel? We can find out; declare a var
to hold the value in the OnGet()
method of Index.cshtml.cs:
var MPAARatings = Request.Query["MPAARatings"];
If we add a breakpoint on this line, and run our code, then check several boxes (you’ll have to continue the first time you hit the breakpoint), then step over the line, we’ll see that the var MPAA
rating is set to a string collection. We could therefore store it in an array property in Index.cshtml.cs, much like we did with our SearchTerms
:
/// <summary>
/// The filtered MPAA Ratings
/// </summary>
public string[] MPAARatings { get; set; }
And we can refactor the line we just added to OnGet()
to use this new property:
MPAARatings = Request.Query["MPAARatings"];
Then, in our Index.cshtml Razor Page, we can refactor the checkbox to be checked if we filtered against this rating in our last request:
<input type="checkbox" name="MPAARatings" value="@rating" checked="@Model.MPAARatings.Contains(rating)"/>
Now our filters stick around when we submit the search request. That just leaves making the filters actually work.
Applying MPAA Rating Filters
Let’s add another method to our MovieDatabase
class, FilterByMPAARating()
:
/// <summary>
/// Filters the provided collection of movies
/// </summary>
/// <param name="movies">The collection of movies to filter</param>
/// <param name="ratings">The ratings to include</param>
/// <returns>A collection containing only movies that match the filter</returns>
public static IEnumerable<Movie> FilterByMPAARating(IEnumerable<Movie> movies, IEnumerable<string> ratings)
{
// TODO: Filter the list
}
Notice that in this method, we accept an IEnumerable<Movie>
parameter. This is the list of movies we want to filter. We use this, instead of the All()
we did in the Search()
method, as we would want to filter the results of a search.
Let’s do a null/empty check, and just return this shortlist if no filters are specified:
// If no filter is specified, just return the provided collection
if (ratings == null || ratings.Count() == 0) return movies;
Otherwise, we’ll use the same process we did before. Start with an empty list of movies, and iterate over the collection seeing if any match. However, as we have two collections (the movies and the ratings), we’ll see if the ratings collection contains the supplied movie’s rating.
// Filter the supplied collection of movies
List<Movie> results = new List<Movie>();
foreach(Movie movie in movies)
{
if(movie.MPAARating != null && ratings.Contains(movie.MPAARating))
{
results.Add(movie);
}
}
Finally, we’ll return our results:
Now, back in our PageModel Index.cshtml.cs, we’ll apply our filter to the results of our search. The refactored OnGet()
should then be:
public void OnGet()
{
SearchTerms = Request.Query["SearchTerms"];
MPAARatings = Request.Query["MPAARatings"];
Movies = MovieDatabase.Search(SearchTerms);
Movies = MovieDatabase.FilterByMPAARating(Movies, MPAARatings);
}
Now we can run a search with filters applied. For example, searching for the word “Love” and movies that are PG or PG-13 yields:
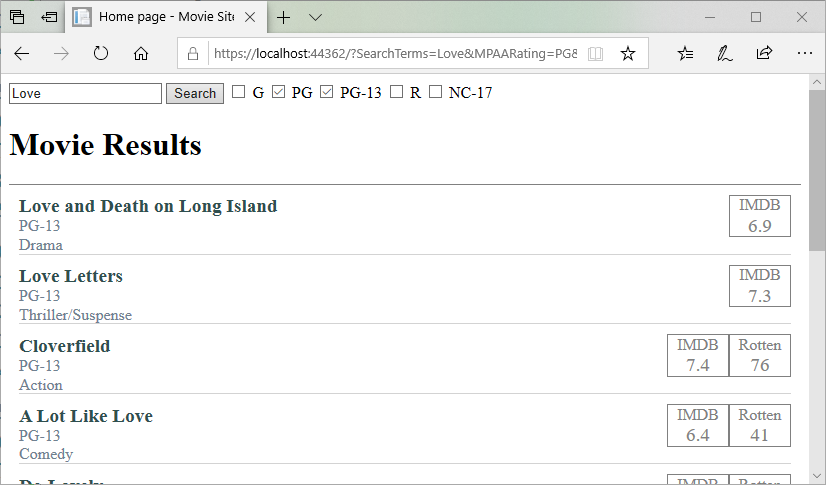
You might be wondering why Cloverfield is listed. But remember, we’re searching by substring, and C LOVE rfield contains love!
Filtering by Genre
Let’s add filters for genre next. But what genres should be included? This is not as clear-cut as our MPAA rating, as there is no standards organization that says “these are the only offical genres that exist.” In fact, new genres emerge from time to time. So a better source of this info might just be to see what Genres are defined in our data, i.e.:
HashSet<string> genres = new HashSet<string>();
foreach(Movie movie in All) {
if(movie.MajorGenre != null)
{
genres.Add(movie.MajorGenre);
}
}
Here we use a HashSet instead of a list, as it only adds each unique item once. Duplicates are ignored.
But where would this code go? We could place it in a getter for MovieDatabase.Genres
:
public IEnumerable<string> Genres
{
get
{
HashSet<string> genres = new HashSet<string>();
foreach(Movie movie in All) {
if(movie.MajorGenre != null)
{
genres.Add(movie.MajorGenre);
}
}
return genres;
}
}
But this means that every time we want to access it, we’ll search through all the movies… This is an O(n) operation, and will make our website slower.
Instead, let’s create a private static variable in the MovieDatabase
class to cache this collection as an array of strings:
// The genres represented in the database
private static string[] _genres;
And expose it with a public static property:
/// <summary>
/// Gets the movie genres represented in the database
/// </summary>
public static string[] Genres => _genres;
And finally, we’ll populate this array in the static constructor of MovieDatabase
, after the JSON file has been processed:
HashSet<string> genreSet = new HashSet<string>();
foreach(Movie movie in _movies) {
if(movie.MajorGenre != null)
{
genreSet.Add(movie.MajorGenre);
}
}
_genres = genreSet.ToArray();
This approach means the finding of genres only happens once, and getting the Genre
property is a constant-time O(1) operation.
We can implement the filters for the genres in the same way as we did for the MPAA filters; I’ll leave that as an exercise for the reader.
Adding Categorical Filters to the Movie Site
Let’s add some filters to the page as well. We’ll start with categorical filters, i.e. filtering by category. Let’s start by filtering for MPAA Rating. We know that there are only a handful of ratings issued by the Motion Picture Association of America - G, PG, PG-13, R, and NC-17. We might be tempted to use an enumeration to represent these values, but in C# an enumeration cannot have strings for values. Nor can we use the hyphen (-
) in an enumeration name.
Defining the MPAA Ratings
So let’s define a string array with our MPAA values, and make it accessible from our MovieDatabase
class:
/// <summary>
/// Gets the possible MPAARatings
/// </summary>
public static string[] MPAARatings
{
get => new string[]
{
"G",
"PG",
"PG-13",
"R",
"NC-17"
};
}
Now in our <form>
in Index.cshtml we can add a checkbox for each of these possible values:
<form>
<input type="text" name="SearchTerms" value="@Model.SearchTerms"/>
<input type="submit" value="Search">
@foreach (string rating in MovieDatabase.MPAARatings)
{
<label>
<input type="checkbox" name="MPAARatings" value="@rating"/>
@rating
</label>
}
</form>
If you try running the project now, and check a few boxes, you’ll see the query string results look something like:
?SearchTerms=&MPAARatings=G&MPAARatings=PG-13
Notice how the key MPAARatings is repeated twice? What would that look like in our PageModel? We can find out; declare a var
to hold the value in the OnGet()
method of Index.cshtml.cs:
var MPAARatings = Request.Query["MPAARatings"];
If we add a breakpoint on this line, and run our code, then check several boxes (you’ll have to continue the first time you hit the breakpoint), then step over the line, we’ll see that the var MPAA
rating is set to a string collection. We could therefore store it in an array property in Index.cshtml.cs, much like we did with our SearchTerms
:
/// <summary>
/// The filtered MPAA Ratings
/// </summary>
public string[] MPAARatings { get; set; }
And we can refactor the line we just added to OnGet()
to use this new property:
MPAARatings = Request.Query["MPAARatings"];
Then, in our Index.cshtml Razor Page, we can refactor the checkbox to be checked if we filtered against this rating in our last request:
<input type="checkbox" name="MPAARatings" value="@rating" checked="@Model.MPAARatings.Contains(rating)"/>
Now our filters stick around when we submit the search request. That just leaves making the filters actually work.
Applying MPAA Rating Filters
Let’s add another method to our MovieDatabase
class, FilterByMPAARating()
:
/// <summary>
/// Filters the provided collection of movies
/// </summary>
/// <param name="movies">The collection of movies to filter</param>
/// <param name="ratings">The ratings to include</param>
/// <returns>A collection containing only movies that match the filter</returns>
public static IEnumerable<Movie> FilterByMPAARating(IEnumerable<Movie> movies, IEnumerable<string> ratings)
{
// TODO: Filter the list
}
Notice that in this method, we accept an IEnumerable<Movie>
parameter. This is the list of movies we want to filter. We use this, instead of the All()
we did in the Search()
method, as we would want to filter the results of a search.
Let’s do a null/empty check, and just return this shortlist if no filters are specified:
// If no filter is specified, just return the provided collection
if (ratings == null || ratings.Count() == 0) return movies;
Otherwise, we’ll use the same process we did before. Start with an empty list of movies, and iterate over the collection seeing if any match. However, as we have two collections (the movies and the ratings), we’ll see if the ratings collection contains the supplied movie’s rating.
// Filter the supplied collection of movies
List<Movie> results = new List<Movie>();
foreach(Movie movie in movies)
{
if(movie.MPAARating != null && ratings.Contains(movie.MPAARating))
{
results.Add(movie);
}
}
Finally, we’ll return our results:
Now, back in our PageModel Index.cshtml.cs, we’ll apply our filter to the results of our search. The refactored OnGet()
should then be:
public void OnGet()
{
SearchTerms = Request.Query["SearchTerms"];
MPAARatings = Request.Query["MPAARatings"];
Movies = MovieDatabase.Search(SearchTerms);
Movies = MovieDatabase.FilterByMPAARating(Movies, MPAARatings);
}
Now we can run a search with filters applied. For example, searching for the word “Love” and movies that are PG or PG-13 yields:
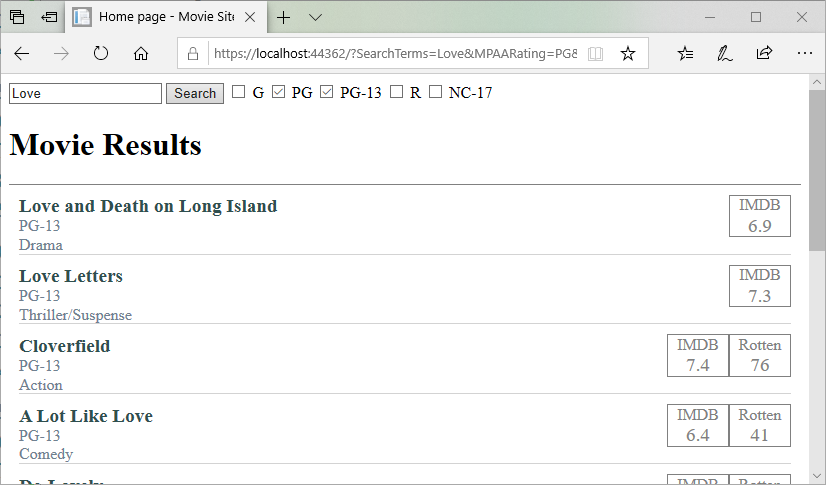
You might be wondering why Cloverfield is listed. But remember, we’re searching by substring, and C LOVE rfield contains love!
Filtering by Genre
Let’s add filters for genre next. But what genres should be included? This is not as clear-cut as our MPAA rating, as there is no standards organization that says “these are the only offical genres that exist.” In fact, new genres emerge from time to time. So a better source of this info might just be to see what Genres are defined in our data, i.e.:
HashSet<string> genres = new HashSet<string>();
foreach(Movie movie in All) {
if(movie.MajorGenre != null)
{
genres.Add(movie.MajorGenre);
}
}
Here we use a HashSet instead of a list, as it only adds each unique item once. Duplicates are ignored.
But where would this code go? We could place it in a getter for MovieDatabase.Genres
:
public static IEnumerable<string> Genres
{
get
{
HashSet<string> genres = new HashSet<string>();
foreach(Movie movie in All) {
if(movie.MajorGenre != null)
{
genres.Add(movie.MajorGenre);
}
}
return genres;
}
}
But this means that every time we want to access it, we’ll search through all the movies… This is an O(n) operation, and will make our website slower.
Instead, let’s create a private static variable in the MovieDatabase
class to cache this collection as an array of strings:
// The genres represented in the database
private static string[] _genres;
And expose it with a public static property:
/// <summary>
/// Gets the movie genres represented in the database
/// </summary>
public static string[] Genres => _genres;
And finally, we’ll populate this array in the static constructor of MovieDatabase
, after the JSON file has been processed:
HashSet<string> genreSet = new HashSet<string>();
foreach(Movie movie in _movies) {
if(movie.MajorGenre != null)
{
genreSet.Add(movie.MajorGenre);
}
}
_genres = genreSet.ToArray();
This approach means the finding of genres only happens once, and getting the Genre
property is a constant-time O(1) operation.
We can implement the filters for the genres in the same way as we did for the MPAA filters; I’ll leave that as an exercise for the reader.
Adding Categorical Filters to the Movie Site
Let’s add some filters to the page as well. We’ll start with categorical filters, i.e. filtering by category. Let’s start by filtering for MPAA Rating. We know that there are only a handful of ratings issued by the Motion Picture Association of America - G, PG, PG-13, R, and NC-17. We might be tempted to use an enumeration to represent these values, but in C# an enumeration cannot have strings for values. Nor can we use the hyphen (-
) in an enumeration name.
Defining the MPAA Ratings
So let’s define a string array with our MPAA values, and make it accessible from our MovieDatabase
class:
/// <summary>
/// Gets the possible MPAARatings
/// </summary>
public static string[] MPAARatings
{
get => new string[]
{
"G",
"PG",
"PG-13",
"R",
"NC-17"
};
}
Now in our <form>
in Index.cshtml we can add a checkbox for each of these possible values:
<form>
@foreach (string rating in MovieDatabase.MPAARatings)
{
<label>
<input type="checkbox" name="MPAARatings" value="@rating"/>
@rating
</label>
}
<input type="text" name="SearchTerms" value="@Model.SearchTerms"/>
<input type="submit" value="Search">
</form>
If you try running the project now, and check a few boxes, you’ll see the query string results look something like:
?SearchTerms=&MPAARatings=G&MPAARatings=PG-13
Notice how the key MPAARatings is repeated twice? What would that look like in our PageModel? We can find out; declare a var
to hold the value in the OnGet()
method of Index.cshtml.cs:
var MPAARatings = Request.Query["MPAARatings"];
If we add a breakpoint on this line, and run our code, then check several boxes (you’ll have to continue the first time you hit the breakpoint), then step over the line, we’ll see that the var MPAA
rating is set to a string collection. We could therefore store it in an array property in Index.cshtml.cs, much like we did with our SearchTerms
:
/// <summary>
/// The filtered MPAA Ratings
/// </summary>
public string[] MPAARatings { get; set; }
And we can refactor the line we just added to OnGet()
to use this new property:
MPAARatings = Request.Query["MPAARatings"];
Then, in our Index.cshtml Razor Page, we can refactor the checkbox to be checked if we filtered against this rating in our last request:
<input type="checkbox" name="MPAARatings" value="@rating" checked="@Model.MPAARatings.Contains(rating)"/>
Now our filters stick around when we submit the search request. That just leaves making the filters actually work.
Applying MPAA Rating Filters
Let’s add another method to our MovieDatabase
class, FilterByMPAARating()
:
/// <summary>
/// Filters the provided collection of movies
/// </summary>
/// <param name="movies">The collection of movies to filter</param>
/// <param name="ratings">The ratings to include</param>
/// <returns>A collection containing only movies that match the filter</returns>
public static IEnumerable<Movie> FilterByMPAARating(IEnumerable<Movie> movies, IEnumerable<string> ratings)
{
// TODO: Filter the list
}
Notice that in this method, we accept an IEnumerable<Movie>
parameter. This is the list of movies we want to filter. We use this, instead of the All()
we did in the Search()
method, as we would want to filter the results of a search.
Let’s do a null/empty check, and just return this shortlist if no filters are specified:
// If no filter is specified, just return the provided collection
if (ratings == null || ratings.Count() == 0) return movies;
Otherwise, we’ll use the same process we did before. Start with an empty list of movies, and iterate over the collection seeing if any match. However, as we have two collections (the movies and the ratings), we’ll see if the ratings collection contains the supplied movie’s rating.
// Filter the supplied collection of movies
List<Movie> results = new List<Movie>();
foreach(Movie movie in movies)
{
if(movie.MPAARatings != null && ratings.Contains(movie.MPAARatings))
{
results.Add(movie);
}
}
Finally, we’ll return our results:
Now, back in our PageModel Index.cshtml.cs, we’ll apply our filter to the results of our search. The refactored OnGet()
should then be:
public void OnGet()
{
SearchTerms = Request.Query["SearchTerms"];
MPAARatings = Request.Query["MPAARatings"];
Movies = MovieDatabase.Search(SearchTerms);
Movies = MovieDatabase.FilterByMPAARating(Movies, MPAARatings);
}
Now we can run a search with filters applied. For example, searching for the word “Love” and movies that are PG or PG-13 yields:
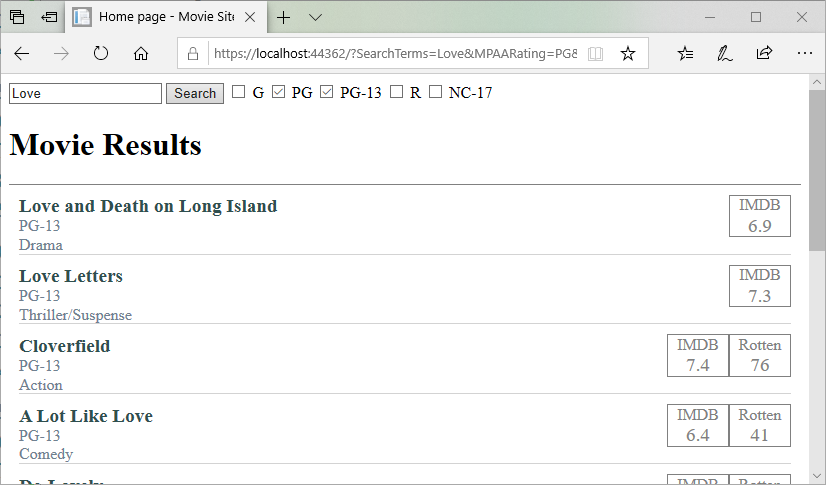
You might be wondering why Cloverfield is listed. But remember, we’re searching by substring, and C LOVE rfield contains love!
Filtering by Genre
Let’s add filters for genre next. But what genres should be included? This is not as clear-cut as our MPAA rating, as there is no standards organization that says “these are the only offical genres that exist.” In fact, new genres emerge from time to time. So a better source of this info might just be to see what Genres are defined in our data, i.e.:
HashSet<string> genres = new HashSet<string>();
foreach(Movie movie in All) {
if(movie.MajorGenre != null)
{
genres.Add(movie.MajorGenre);
}
}
Here we use a HashSet instead of a list, as it only adds each unique item once. Duplicates are ignored.
But where would this code go? We could place it in a getter for MovieDatabase.Genres
:
public IEnumerable<string> Genres
{
get
{
HashSet<string> genres = new HashSet<string>();
foreach(Movie movie in All) {
if(movie.MajorGenre != null)
{
genres.Add(movie.MajorGenre);
}
}
}
}
But this means that every time we want to access it, we’ll search through all the movies… This is an O(n) operation, and will make our website slower.
Instead, let’s create a private static variable in the MovieDatabase
class to cache this collection as an array of strings:
// The genres represented in the database
private static string[] _genres;
And expose it with a public static property:
/// <summary>
/// Gets the movie genres represented in the database
/// </summary>
public static string[] Genres => _genres;
And finally, we’ll populate this array in the static constructor of MovieDatabase
, after the JSON file has been processed:
HashSet<string> genreSet = new HashSet<string>();
foreach(Movie movie in _movies) {
if(movie.MajorGenre != null)
{
genreSet.Add(movie.MajorGenre);
}
}
_genres = genreSet.ToArray();
This approach means the finding of genres only happens once, and getting the Genre
property is a constant-time O(1) operation.
We can implement the filters for the genres in the same way as we did for the MPAA filters; I’ll leave that as an exercise for the reader.
Numerical Filters
Let’s tackle one of the critics ratings next. While we could create categories and use checkboxes, this doesn’t capture the incremental values (i.e. 4.3), and it would be a lot of checkboxes for Rotten Tomatoes ratings! Instead, we’ll use a numerical filter, which limits our possible results to a range - between a minimum and maximum value.
Moreover, let’s clean up our Index page, as it is getting difficult to determine what filter(s) go together, and and are adding more.
Refactoring the Index Page
Let’s move the filters to a column on the left, leave the search bar above, and show our results on the right. This will require refactoring our Index.cshtml file:
<form id="movie-database">
<div id="search">
<input type="text" name="SearchTerms" value="@Model.SearchTerms" />
<input type="submit" value="Search">
</div>
<div id="filters">
<h4>MPAA Rating</h4>
@foreach (string rating in MovieDatabase.MPAARating)
{
<label>
<input type="checkbox" name="MPAARatings" value="@rating" checked="@Model.MPAARatings.Contains(rating)" />
@rating
</label>
}
<h4>Genre</h4>
@foreach (string genre in MovieDatabase.Genres)
{
<label>
<input type="checkbox" name="Genres" value="@genre" />
@genre
</label>
}
<h4>IMDB Rating</h4>
<div>
Between
<input name="IMDBMin" type="number" min="0" max="10" step="0.1" placeholder="min"/>
and
<input name="IMDBMax" type="number" min="0" max="10" step="0.1" placeholder="max"/>
</div>
</div>
<div id="results">
<h1>Movie Results</h1>
<ul class="movie-list">
@foreach (Movie movie in @Model.Movies)
{
<li>
<div class="details">
<h3 class="title">@movie.Title</h3>
<div class="mpaa">@movie.MPAARating</div>
<div class="genre">@movie.MajorGenre</div>
</div>
<div class="ratings">
@if (movie.IMDBRating != null)
{
<div class="imdb">
@movie.IMDBRating
</div>
}
@if (movie.RottenTomatoesRating != null)
{
<div class="rotten-tomatoes">
@movie.RottenTomatoesRating
</div>
}
</div>
</li>
}
</ul>
</div>
</form>
Most of this is simply moving elements around the page, but note that we are using inputs of type=number
to represent our range of IMDB values. We can specify a minimum and maximum for this range, as well as an allowable increment. Also, we use the placeholder
attribute to put text into the input
until a value is added.
Adding More Styles
Now we’ll need to add some rules to our wwwroot/css/styles.css. First, we’ll use a grid
for the layout of the form:
form#movie-database {
display: grid;
grid-template-columns: 1fr 3fr;
grid-template-rows: auto auto;
}
The right column will be three times as big as the right.
We can make our search bar span both columns with grid-column-start
and grid-column-end
:
#search {
grid-column-start: 1;
grid-column-end: 3;
text-align: center;
}
Notice too that for CSS, we start counting at 1, not 0. The filters and the results will fall in the next row automatically, each taking up their own respective grid cell. You can read more about the grid layout in A Complete Guide to Grid.
Let’s go ahead and use flexbox to lay out our filters in a column:
#filters {
display: flex;
flex-direction: column;
}
And make our number inputs bigger:
#filters input[type=number] {
width: 4rem;
}
Notice the use of square brackets in our CSS Selector to only apply to inputs with type number.
Also, let’s add a margin above and remove most of the margin below our <h4>
elements:
#filters h4 {
margin-bottom: 0.2rem;
margin-top: 2rem;
}
The resulting page looks much cleaner:
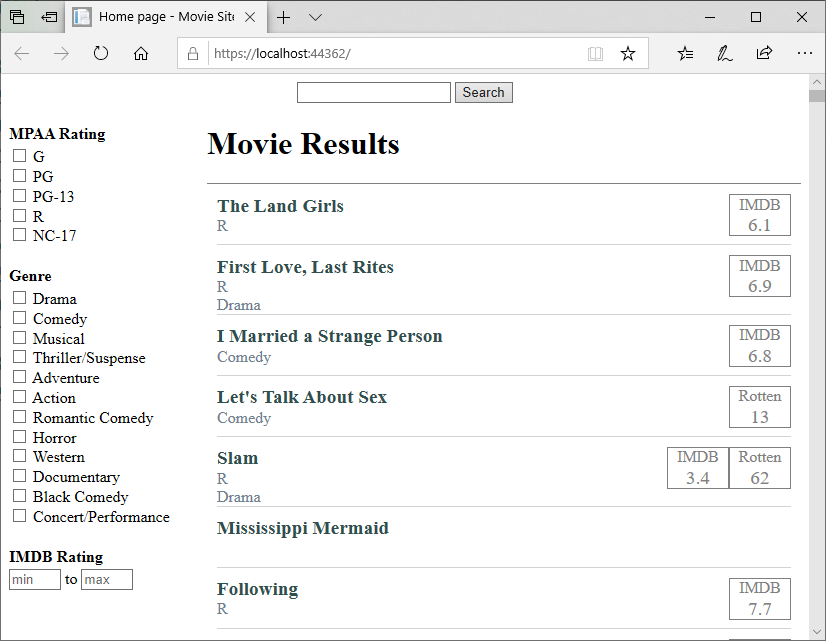
Capturing the Filter Values
Now we need to get the filter values from our GET request query string. We could do this like we’ve been doing before, with:
Request.Query["IMDBMin"];
But the returned value would be a string, so we’d need to parse it:
IMDBMin = double.Parse(Request.Query["IMDBMin"]);
If the query was null
, then this would evaluate to NaN
, which we wouldn’t want to set our <input>
to…
Instead, we’ll look at some options built into the PageModel.
Parameter Binding
The first of these options is Parameter Binding. In this approach, we define parameters to our OnGet()
method to be parsed out of the request automatically, i.e.:
/// <summary>
/// Gets the search results for display on the page
/// </summary>
public void OnGet(string SearchTerms, string[] MPAARatings, string[] Genres, double? IMDBMin, double? IMDBMax) {
this.SearchTerms = SearchTerms;
this.MPAARatings = MPAARatings;
this.Genres = Genres;
this.IMDBMin = IMDBMin;
this.IMDBMax = IMDBMax;
Movies = MovieDatabase.Search(SearchTerms);
Movies = MovieDatabase.FilterByMPAARating(Movies, MPAARatings);
Movies = MovieDatabase.FilterByGenre(Movies, Genres);
Movies = MovieDatabase.FilterByIMDBRating(Movies, IMDBMin, IMDBMax);
}
The benefit of this approach is that as long as C# knows a conversion into the type we specify, the conversion is done automatically. Note that the parameter name matches the name
property of the corresponding <input>
- this must be the case for the Razor Page to bind the parameter to the corresponding input value.
Note that we still need to assign these parameter values to the corresponding properties of our PageModel. If we don’t, then those properties will all be null
, and the <inputs>
rendered on our page will always be blank.
Model Binding
A second option is to use Model Binding. Model binding also automatically converts incoming form data, but in this case it binds directly to the properties of our PageModel. We indicate this form of binding with a [BindProperty]
attribute, i.e.:
public class IndexModel : PageModel {
[BindProperty(SupportsGet=true)]
public string SearchTerms {get; set;}
[BindProperty(SupportsGet=true)]
public string[] MPAARatings {get; set;}
[BindProperty(SupportsGet=true)]
public string[] Genres {get; set;}
[BindProperty(SupportsGet=true)]
public double? IMDBMin {get; set;}
[BindProperty(SupportsGet=true)]
public double? IMDBMax {get; set;}
/// <summary>
/// Gets the search results for display on the page
/// </summary>
public void OnGet() {
Movies = MovieDatabase.Search(SearchTerms);
Movies = MovieDatabase.FilterByMPAARating(Movies, MPAARatings);
Movies = MovieDatabase.FilterByGenre(Movies, Genres);
Movies = MovieDatabase.FilterByIMDBRating(Movies, IMDBMin, IMDBMax);
}
}
Note that with this approach, the incoming data is directly bound to the properties, so we don’t need to do any special assignments within our OnGet()
method. Also, note that we have to use SupportsGet=true
in order for this binding to occur on GET requests (by default, model binding only happens with POST requests).
Note
You only need to do one binding approach per property in a PageModel. I.e. you can just use the property decorator:
public class SomePageModel : PageModel
{
[BindProperty(SupportsGet=true)]
public float SomeProperty { get; set; }
public void OnGet() {
DoSomething(SomeProperty);
}
}
or you might use parameter binding:
public class SomePageModel : PageModel
{
public void OnGet(float SomeProperty) {
DoSomething(SomeProperty);
}
}
or you can parse it from the request:
public class SomePageModel : PageModel
{
public void OnGet() {
var someProperty = float.Parse(Request.Query["SomeProperty"]);
DoSomething(someProperty);
}
}
These are all different means of accessing the same data from the incoming request.
Now all we need to do is implement the actual filter.
Implementing the IMDB Rating Filter
We’ll define the new filter in our MovieDatabase
class as another static method:
/// <summary>
/// Filters the provided collection of movies
/// to those with IMDB ratings falling within
/// the specified range
/// </summary>
/// <param name="movies">The collection of movies to filter</param>
/// <param name="min">The minimum range value</param>
/// <param name="max">The maximum range value</param>
/// <returns>The filtered movie collection</returns>
public static IEnumerable<Movie> FilterByIMDBRating(IEnumerable<Movie> movies, double? min, double? max)
{
// TODO: Filter movies
}
Notice that here too we use the nullable double value. So our first step is probably to do a null
check:
if (min == null && max == null) return movies;
But what if only one is null? Should we filter for that part of the range? It wouldn’t be hard to do:
var results = new List<Movie>();
// only a maximum specified
if(min == null)
{
foreach(Movie movie in movies)
{
if (movie.IMDBRating <= max) results.Add(movie);
}
return results;
}
And the minimum would mirror that:
// only a minimum specified
if(max == null)
{
foreach(Movie movie in movies)
{
if (movie.IMDBRating >= min) results.Add(movie);
}
return results;
}
Finally, we could handle the case where we have both a min and max value to our range:
// Both minimum and maximum specified
foreach(Movie movie in movies)
{
if(movie.IMDBRating >= min && movie.IMDBRating <= max)
{
results.Add(movie);
}
}
return results;
Notice too, that in each of these cases we’re treating the range as inclusive (including the specified minimum and maximum). This is the behavior most casual internet users will expect. If the database and user expectations are different for your audience, you’d want your code to match that expectation.
Now we can filter by IMDB rating:
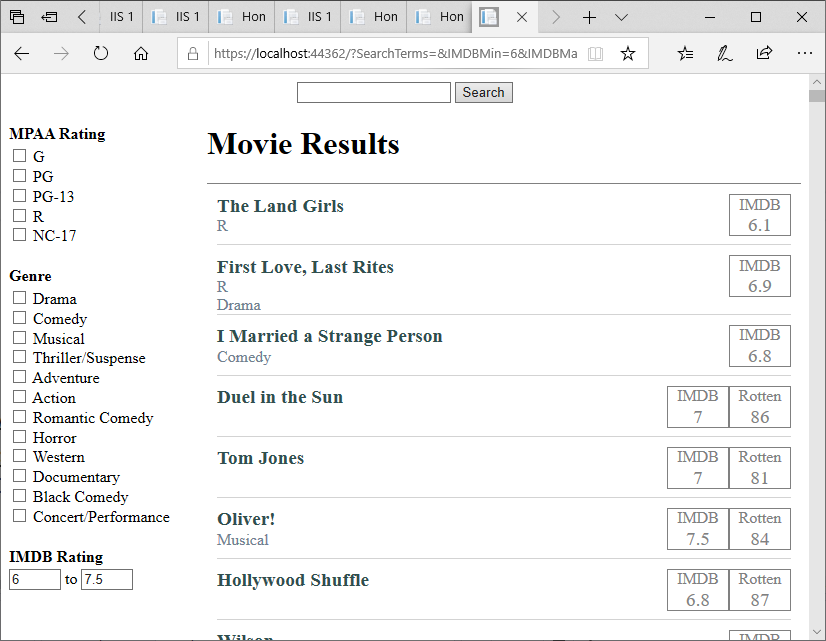
Finishing Up
Since we’re displaying the Rotten Tomatoes rating, we should probably also have a filter for it. This will work almost exactly like the IMDB rating - but with the range from 0 to 100. I’ll leave this as an exercise for the reader.
Numerical Filters
Let’s tackle one of the critics ratings next. While we could create categories and use checkboxes, this doesn’t capture the incremental values (i.e. 4.3), and it would be a lot of checkboxes for Rotten Tomatoes ratings! Instead, we’ll use a numerical filter, which limits our possible results to a range - between a minimum and maximum value.
Moreover, let’s clean up our Index page, as it is getting difficult to determine what filter(s) go together, and and are adding more.
Refactoring the Index Page
Let’s move the filters to a column on the left, leave the search bar above, and show our results on the right. This will require refactoring our Index.cshtml file:
<form id="movie-database">
<div id="search">
<input type="text" name="SearchTerms" value="@Model.SearchTerms" />
<input type="submit" value="Search">
</div>
<div id="filters">
<h4>MPAA Rating</h4>
@foreach (string rating in MovieDatabase.MPAARating)
{
<label>
<input type="checkbox" name="MPAARatings" value="@rating" checked="@Model.MPAARatings.Contains(rating)" />
@rating
</label>
}
<h4>Genre</h4>
@foreach (string genre in MovieDatabase.Genres)
{
<label>
<input type="checkbox" name="Genres" value="@genre" />
@genre
</label>
}
<h4>IMDB Rating</h4>
<div>
Between
<input name="IMDBMin" type="number" min="0" max="10" step="0.1" placeholder="min"/>
and
<input name="IMDBMax" type="number" min="0" max="10" step="0.1" placeholder="max"/>
</div>
</div>
<div id="results">
<h1>Movie Results</h1>
<ul class="movie-list">
@foreach (Movie movie in @Model.Movies)
{
<li>
<div class="details">
<h3 class="title">@movie.Title</h3>
<div class="mpaa">@movie.MPAARating</div>
<div class="genre">@movie.MajorGenre</div>
</div>
<div class="ratings">
@if (movie.IMDBRating != null)
{
<div class="imdb">
@movie.IMDBRating
</div>
}
@if (movie.RottenTomatoesRating != null)
{
<div class="rotten-tomatoes">
@movie.RottenTomatoesRating
</div>
}
</div>
</li>
}
</ul>
</div>
</form>
Most of this is simply moving elements around the page, but note that we are using inputs of type=number
to represent our range of IMDB values. We can specify a minimum and maximum for this range, as well as an allowable increment. Also, we use the placeholder
attribute to put text into the input
until a value is added.
Adding More Styles
Now we’ll need to add some rules to our wwwroot/css/styles.css. First, we’ll use a grid
for the layout of the form:
form#movie-database {
display: grid;
grid-template-columns: 1fr 3fr;
grid-template-rows: auto auto;
}
The right column will be three times as big as the left.
We can make our search bar span both columns with grid-column-start
and grid-column-end
:
#search {
grid-column-start: 1;
grid-column-end: 3;
text-align: center;
}
Notice too that for CSS, we start counting at 1, not 0. The filters and the results will fall in the next row automatically, each taking up their own respective grid cell. You can read more about the grid layout in A Complete Guide to Grid.
Let’s go ahead and use flexbox to lay out our filters in a column:
#filters {
display: flex;
flex-direction: column;
}
And make our number inputs bigger:
#filters input[type=number] {
width: 4rem;
}
Notice the use of square brackets in our CSS Selector to only apply to inputs with type number.
Also, let’s add a margin above and remove most of the margin below our <h4>
elements:
#filters h4 {
margin-bottom: 0.2rem;
margin-top: 2rem;
}
The resulting page looks much cleaner:
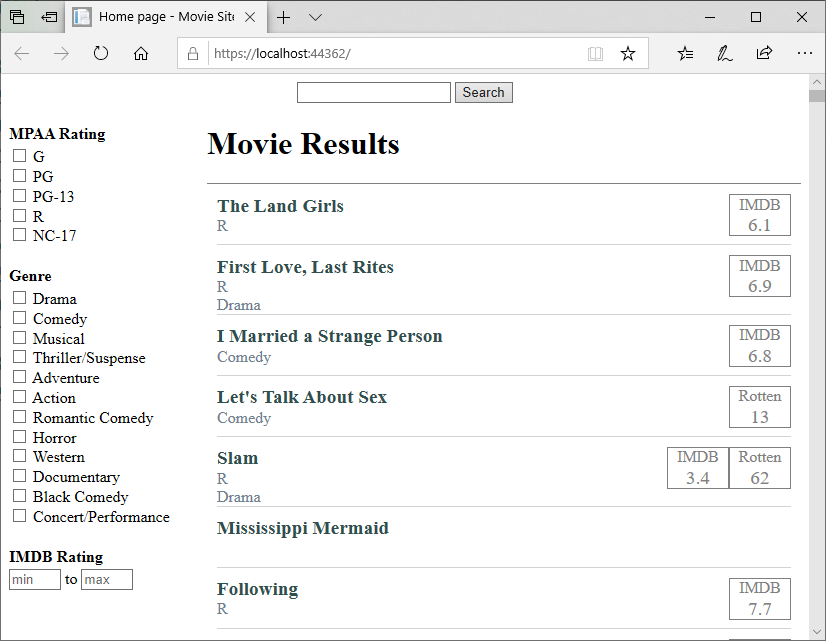
Capturing the Filter Values
Now we need to get the filter values from our GET request query string. We could do this like we’ve been doing before, with:
Request.Query["IMDBMin"];
But the returned value would be a string, so we’d need to parse it:
IMDBMin = double.Parse(Request.Query["IMDBMin"]);
If the query was null
, then this would evaluate to NaN
, which we wouldn’t want to set our <input>
to…
Instead, we’ll look at some options built into the PageModel.
Parameter Binding
The first of these options is Parameter Binding. In this approach, we define parameters to our OnGet()
method to be parsed out of the request automatically, i.e.:
/// <summary>
/// Gets the search results for display on the page
/// </summary>
public void OnGet(string SearchTerms, string[] MPAARatings, string[] Genres, double? IMDBMin, double? IMDBMax) {
this.SearchTerms = SearchTerms;
this.MPAARatings = MPAARatings;
this.Genres = Genres;
this.IMDBMin = IMDBMin;
this.IMDBMax = IMDBMax;
Movies = MovieDatabase.Search(SearchTerms);
Movies = MovieDatabase.FilterByMPAARating(Movies, MPAARatings);
Movies = MovieDatabase.FilterByGenre(Movies, Genres);
Movies = MovieDatabase.FilterByIMDBRating(Movies, IMDBMin, IMDBMax);
}
The benefit of this approach is that as long as C# knows a conversion into the type we specify, the conversion is done automatically. Note that the parameter name matches the name
property of the corresponding <input>
- this must be the case for the Razor Page to bind the parameter to the corresponding input value.
Note that we still need to assign these parameter values to the corresponding properties of our PageModel. If we don’t, then those properties will all be null
, and the <inputs>
rendered on our page will always be blank.
Model Binding
A second option is to use Model Binding. Model binding also automatically converts incoming form data, but in this case it binds directly to the properties of our PageModel. We indicate this form of binding with a [BindProperty]
attribute, i.e.:
public class IndexModel : PageModel {
[BindProperty(SupportsGet=true)]
public string SearchTerms {get; set;}
[BindProperty(SupportsGet=true)]
public string[] MPAARatings {get; set;}
[BindProperty(SupportsGet=true)]
public string[] Genres {get; set;}
[BindProperty(SupportsGet=true)]
public double? IMDBMin {get; set;}
[BindProperty(SupportsGet=true)]
public double? IMDBMax {get; set;}
/// <summary>
/// Gets the search results for display on the page
/// </summary>
public void OnGet() {
Movies = MovieDatabase.Search(SearchTerms);
Movies = MovieDatabase.FilterByMPAARating(Movies, MPAARatings);
Movies = MovieDatabase.FilterByGenre(Movies, Genres);
Movies = MovieDatabase.FilterByIMDBRating(Movies, IMDBMin, IMDBMax);
}
}
Note that with this approach, the incoming data is directly bound to the properties, so we don’t need to do any special assignments within our OnGet()
method. Also, note that we have to use SupportsGet=true
in order for this binding to occur on GET requests (by default, model binding only happens with POST requests).
Note
You only need to do one binding approach per property in a PageModel. I.e. you can just use the property decorator:
public class SomePageModel : PageModel
{
[BindProperty(SupportsGet=true)]
public float SomeProperty { get; set; }
public void OnGet() {
DoSomething(SomeProperty);
}
}
or you might use parameter binding:
public class SomePageModel : PageModel
{
public void OnGet(float SomeProperty) {
DoSomething(SomeProperty);
}
}
or you can parse it from the request:
public class SomePageModel : PageModel
{
public void OnGet() {
var someProperty = float.Parse(Request.Query["SomeProperty"]);
DoSomething(someProperty);
}
}
These are all different means of accessing the same data from the incoming request.
Now all we need to do is implement the actual filter.
Implementing the IMDB Rating Filter
We’ll define the new filter in our MovieDatabase
class as another static method:
/// <summary>
/// Filters the provided collection of movies
/// to those with IMDB ratings falling within
/// the specified range
/// </summary>
/// <param name="movies">The collection of movies to filter</param>
/// <param name="min">The minimum range value</param>
/// <param name="max">The maximum range value</param>
/// <returns>The filtered movie collection</returns>
public static IEnumerable<Movie> FilterByIMDBRating(IEnumerable<Movie> movies, double? min, double? max)
{
// TODO: Filter movies
}
Notice that here too we use the nullable double value. So our first step is probably to do a null
check:
if (min == null && max == null) return movies;
But what if only one is null? Should we filter for that part of the range? It wouldn’t be hard to do:
var results = new List<Movie>();
// only a maximum specified
if(min == null)
{
foreach(Movie movie in movies)
{
if (movie.IMDBRating <= max) results.Add(movie);
}
return results;
}
And the minimum would mirror that:
// only a minimum specified
if(max == null)
{
foreach(Movie movie in movies)
{
if (movie.IMDBRating >= min) results.Add(movie);
}
return results;
}
Finally, we could handle the case where we have both a min and max value to our range:
// Both minimum and maximum specified
foreach(Movie movie in movies)
{
if(movie.IMDBRating >= min && movie.IMDBRating <= max)
{
results.Add(movie);
}
}
return results;
Notice too, that in each of these cases we’re treating the range as inclusive (including the specified minimum and maximum). This is the behavior most casual internet users will expect. If the database and user expectations are different for your audience, you’d want your code to match that expectation.
Now we can filter by IMDB rating:
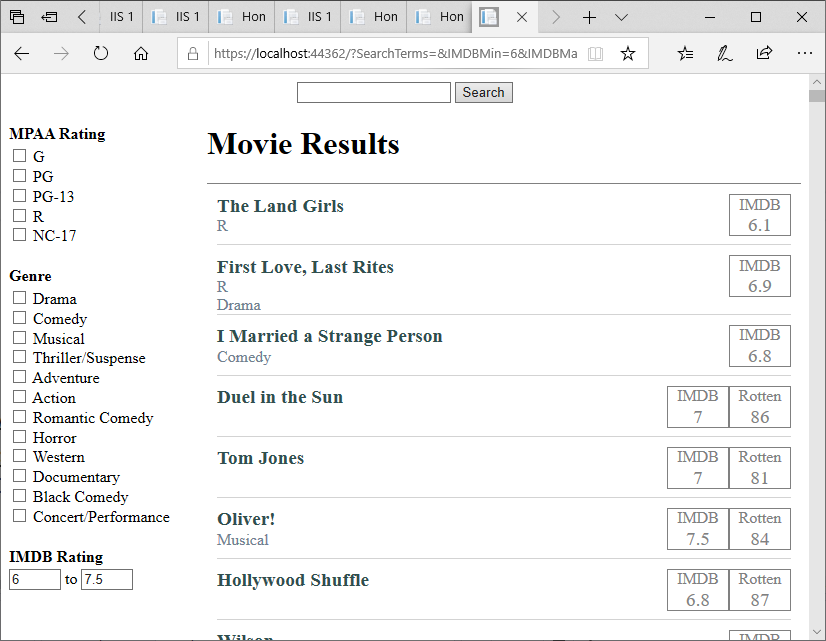
Finishing Up
Since we’re displaying the Rotten Tomatoes rating, we should probably also have a filter for it. This will work almost exactly like the IMDB rating - but with the range from 0 to 100. I’ll leave this as an exercise for the reader.
Numerical Filters
Let’s tackle one of the critics ratings next. While we could create categories and use checkboxes, this doesn’t capture the incremental values (i.e. 4.3), and it would be a lot of checkboxes for Rotten Tomatoes ratings! Instead, we’ll use a numerical filter, which limits our possible results to a range - between a minimum and maximum value.
Moreover, let’s clean up our Index page, as it is getting difficult to determine what filter(s) go together, and and are adding more.
Refactoring the Index Page
Let’s move the filters to a column on the left, leave the search bar above, and show our results on the right. This will require refactoring our Index.cshtml file:
<form id="movie-database">
<div id="search">
<input type="text" name="SearchTerms" value="@Model.SearchTerms" />
<input type="submit" value="Search">
</div>
<div id="filters">
<h4>MPAA Rating</h4>
@foreach (string rating in MovieDatabase.MPAARatings)
{
<label>
<input type="checkbox" name="MPAARatings" value="@rating" checked="@Model.MPAARatings.Contains(rating)" />
@rating
</label>
}
<h4>Genre</h4>
@foreach (string genre in MovieDatabase.Genres)
{
<label>
<input type="checkbox" name="Genres" value="@genre" />
@genre
</label>
}
<h4>IMDB Rating</h4>
<div>
Between
<input name="IMDBMin" type="number" min="0" max="10" step="0.1" placeholder="min"/>
and
<input name="IMDBMax" type="number" min="0" max="10" step="0.1" placeholder="max"/>
</div>
</div>
<div id="results">
<h1>Movie Results</h1>
<ul class="movie-list">
@foreach (Movie movie in @Model.Movies)
{
<li>
<div class="details">
<h3 class="title">@movie.Title</h3>
<div class="mpaa">@movie.MPAARating</div>
<div class="genre">@movie.MajorGenre</div>
</div>
<div class="ratings">
@if (movie.IMDBRating != null)
{
<div class="imdb">
@movie.IMDBRating
</div>
}
@if (movie.RottenTomatoesRating != null)
{
<div class="rotten-tomatoes">
@movie.RottenTomatoesRating
</div>
}
</div>
</li>
}
</ul>
</div>
</form>
Most of this is simply moving elements around the page, but note that we are using inputs of type=number
to represent our range of IMDB values. We can specify a minimum and maximum for this range, as well as an allowable increment. Also, we use the placeholder
attribute to put text into the input
until a value is added.
Adding More Styles
Now we’ll need to add some rules to our wwwroot/css/styles.css. First, we’ll use a grid
for the layout of the form:
form#movie-database {
display: grid;
grid-template-columns: 1fr 3fr;
grid-template-rows: auto auto;
}
The right column will be three times as big as the left.
We can make our search bar span both columns with grid-column-start
and grid-column-end
:
#search {
grid-column-start: 1;
grid-column-end: 3;
text-align: center;
}
Notice too that for CSS, we start counting at 1, not 0. The filters and the results will fall in the next row automatically, each taking up their own respective grid cell. You can read more about the grid layout in A Complete Guide to Grid.
Let’s go ahead and use flexbox to lay out our filters in a column:
#filters {
display: flex;
flex-direction: column;
}
And make our number inputs bigger:
#filters input[type=number] {
width: 4rem;
}
Notice the use of square brackets in our CSS Selector to only apply to inputs with type number.
Also, let’s add a margin above and remove most of the margin below our <h4>
elements:
#filters h4 {
margin-bottom: 0.2rem;
margin-top: 2rem;
}
The resulting page looks much cleaner:
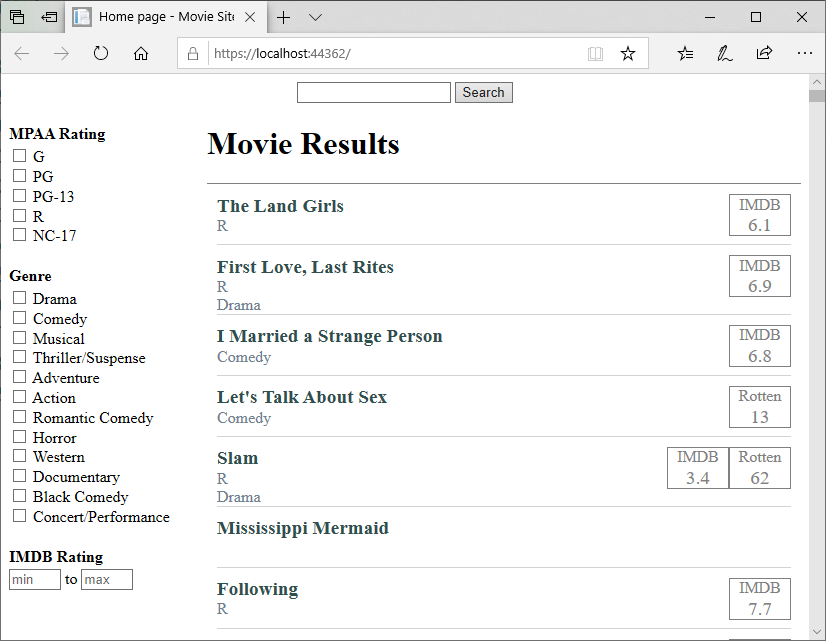
Capturing the Filter Values
Now we need to get the filter values from our GET request query string. We could do this like we’ve been doing before, with:
Request.Query["IMDBMin"];
But the returned value would be a string, so we’d need to parse it:
IMDBMin = double.Parse(Request.Query["IMDBMin"]);
If the query was null
, then this would evaluate to NaN
, which we wouldn’t want to set our <input>
to…
Instead, we’ll look at some options built into the PageModel.
Parameter Binding
The first of these options is Parameter Binding. In this approach, we define parameters to our OnGet()
method to be parsed out of the request automatically, i.e.:
/// <summary>
/// Gets the search results for display on the page
/// </summary>
public void OnGet(string SearchTerms, string[] MPAARatings, string[] Genres, double? IMDBMin, double? IMDBMax) {
this.SearchTerms = SearchTerms;
this.MPAARatings = MPAARatings;
this.Genres = Genres;
this.IMDBMin = IMDBMin;
this.IMDBMax = IMDBMax;
Movies = MovieDatabase.Search(SearchTerms);
Movies = MovieDatabase.FilterByMPAARating(Movies, MPAARatings);
Movies = MovieDatabase.FilterByGenre(Movies, Genres);
Movies = MovieDatabase.FilterByIMDBRating(Movies, IMDBMin, IMDBMax);
}
The benefit of this approach is that as long as C# knows a conversion into the type we specify, the conversion is done automatically. Note that the parameter name matches the name
property of the corresponding <input>
- this must be the case for the Razor Page to bind the parameter to the corresponding input value.
Note that we still need to assign these parameter values to the corresponding properties of our PageModel. If we don’t, then those properties will all be null
, and the <inputs>
rendered on our page will always be blank.
Model Binding
A second option is to use Model Binding. Model binding also automatically converts incoming form data, but in this case it binds directly to the properties of our PageModel. We indicate this form of binding with a [BindProperty]
attribute, i.e.:
public class IndexModel : PageModel {
[BindProperty(SupportsGet=true)]
public string SearchTerms {get; set;}
[BindProperty(SupportsGet=true)]
public string[] MPAARatings {get; set;}
[BindProperty(SupportsGet=true)]
public string[] Genres {get; set;}
[BindProperty(SupportsGet=true)]
public double? IMDBMin {get; set;}
[BindProperty(SupportsGet=true)]
public double? IMDBMax {get; set;}
/// <summary>
/// Gets the search results for display on the page
/// </summary>
public void OnGet() {
Movies = MovieDatabase.Search(SearchTerms);
Movies = MovieDatabase.FilterByMPAARating(Movies, MPAARatings);
Movies = MovieDatabase.FilterByGenre(Movies, Genres);
Movies = MovieDatabase.FilterByIMDBRating(Movies, IMDBMin, IMDBMax);
}
}
Note that with this approach, the incoming data is directly bound to the properties, so we don’t need to do any special assignments within our OnGet()
method. Also, note that we have to use SupportsGet=true
in order for this binding to occur on GET requests (by default, model binding only happens with POST requests).
Note
You only need to do one binding approach per property in a PageModel. I.e. you can just use the property decorator:
public class SomePageModel : PageModel
{
[BindProperty(SupportsGet=true)]
public float SomeProperty { get; set; }
public void OnGet() {
DoSomething(SomeProperty);
}
}
or you might use parameter binding:
public class SomePageModel : PageModel
{
public void OnGet(float SomeProperty) {
DoSomething(SomeProperty);
}
}
or you can parse it from the request:
public class SomePageModel : PageModel
{
public void OnGet() {
var someProperty = float.Parse(Request.Query["SomeProperty"]);
DoSomething(someProperty);
}
}
These are all different means of accessing the same data from the incoming request.
Now all we need to do is implement the actual filter.
Implementing the IMDB Rating Filter
We’ll define the new filter in our MovieDatabase
class as another static method:
/// <summary>
/// Filters the provided collection of movies
/// to those with IMDB ratings falling within
/// the specified range
/// </summary>
/// <param name="movies">The collection of movies to filter</param>
/// <param name="min">The minimum range value</param>
/// <param name="max">The maximum range value</param>
/// <returns>The filtered movie collection</returns>
public static IEnumerable<Movie> FilterByIMDBRating(IEnumerable<Movie> movies, double? min, double? max)
{
// TODO: Filter movies
}
Notice that here too we use the nullable double value. So our first step is probably to do a null
check:
if (min == null && max == null) return movies;
But what if only one is null? Should we filter for that part of the range? It wouldn’t be hard to do:
var results = new List<Movie>();
// only a maximum specified
if(min == null)
{
foreach(Movie movie in movies)
{
if (movie.IMDBRating <= max) results.Add(movie);
}
return results;
}
And the minimum would mirror that:
// only a minimum specified
if(max == null)
{
foreach(Movie movie in movies)
{
if (movie.IMDBRating >= min) results.Add(movie);
}
return results;
}
Finally, we could handle the case where we have both a min and max value to our range:
// Both minimum and maximum specified
foreach(Movie movie in movies)
{
if(movie.IMDBRating >= min && movie.IMDBRating <= max)
{
results.Add(movie);
}
}
return results;
Notice too, that in each of these cases we’re treating the range as inclusive (including the specified minimum and maximum). This is the behavior most casual internet users will expect. If the database and user expectations are different for your audience, you’d want your code to match that expectation.
Now we can filter by IMDB rating:
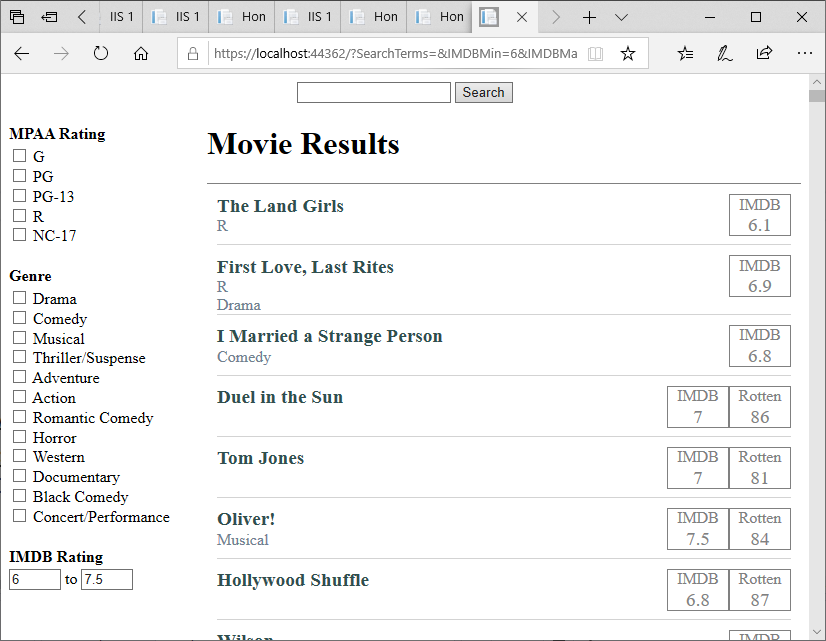
Finishing Up
Since we’re displaying the Rotten Tomatoes rating, we should probably also have a filter for it. This will work almost exactly like the IMDB rating - but with the range from 0 to 100. I’ll leave this as an exercise for the reader.
Numerical Filters
Let’s tackle one of the critics ratings next. While we could create categories and use checkboxes, this doesn’t capture the incremental values (i.e. 4.3), and it would be a lot of checkboxes for Rotten Tomatoes ratings! Instead, we’ll use a numerical filter, which limits our possible results to a range - between a minimum and maximum value.
Moreover, let’s clean up our Index page, as it is getting difficult to determine what filter(s) go together, and and are adding more.
Refactoring the Index Page
Let’s move the filters to a column on the left, leave the search bar above, and show our results on the right. This will require refactoring our Index.cshtml file:
<form id="movie-database">
<div id="search">
<input type="text" name="SearchTerms" value="@Model.SearchTerms" />
<input type="submit" value="Search">
</div>
<div id="filters">
<h4>MPAA Rating</h4>
@foreach (string rating in MovieDatabase.MPAARatings)
{
<label>
<input type="checkbox" name="MPAARatings" value="@rating" checked="@Model.MPAARatings.Contains(rating)" />
@rating
</label>
}
<h4>Genre</h4>
@foreach (string genre in MovieDatabase.Genres)
{
<label>
<input type="checkbox" name="Genres" value="@genre" />
@genre
</label>
}
<h4>IMDB Rating</h4>
<div>
Between
<input name="IMDBMin" type="number" min="0" max="10" step="0.1" placeholder="min"/>
and
<input name="IMDBMax" type="number" min="0" max="10" step="0.1" placeholder="max"/>
</div>
</div>
<div id="results">
<h1>Movie Results</h1>
<ul class="movie-list">
@foreach (Movie movie in @Model.Movies)
{
<li>
<div class="details">
<h3 class="title">@movie.Title</h3>
<div class="mpaa">@movie.MPAARating</div>
<div class="genre">@movie.MajorGenre</div>
</div>
<div class="ratings">
@if (movie.IMDBRating != null)
{
<div class="imdb">
@movie.IMDBRating
</div>
}
@if (movie.RottenTomatoesRating != null)
{
<div class="rotten-tomatoes">
@movie.RottenTomatoesRating
</div>
}
</div>
</li>
}
</ul>
</div>
</form>
Most of this is simply moving elements around the page, but note that we are using inputs of type=number
to represent our range of IMDB values. We can specify a minimum and maximum for this range, as well as an allowable increment. Also, we use the placeholder
attribute to put text into the input
until a value is added.
Adding More Styles
Now we’ll need to add some rules to our wwwroot/css/styles.css. First, we’ll use a grid
for the layout of the form:
form#movie-database {
display: grid;
grid-template-columns: 1fr 3fr;
grid-template-rows: auto auto;
}
The right column will be three times as big as the left.
We can make our search bar span both columns with grid-column-start
and grid-column-end
:
#search {
grid-column-start: 1;
grid-column-end: 3;
text-align: center;
}
Notice too that for CSS, we start counting at 1, not 0. The filters and the results will fall in the next row automatically, each taking up their own respective grid cell. You can read more about the grid layout in A Complete Guide to Grid.
Let’s go ahead and use flexbox to lay out our filters in a column:
#filters {
display: flex;
flex-direction: column;
}
And make our number inputs bigger:
#filters input[type=number] {
width: 4rem;
}
Notice the use of square brackets in our CSS Selector to only apply to inputs with type number.
Also, let’s add a margin above and remove most of the margin below our <h4>
elements:
#filters h4 {
margin-bottom: 0.2rem;
margin-top: 2rem;
}
The resulting page looks much cleaner:
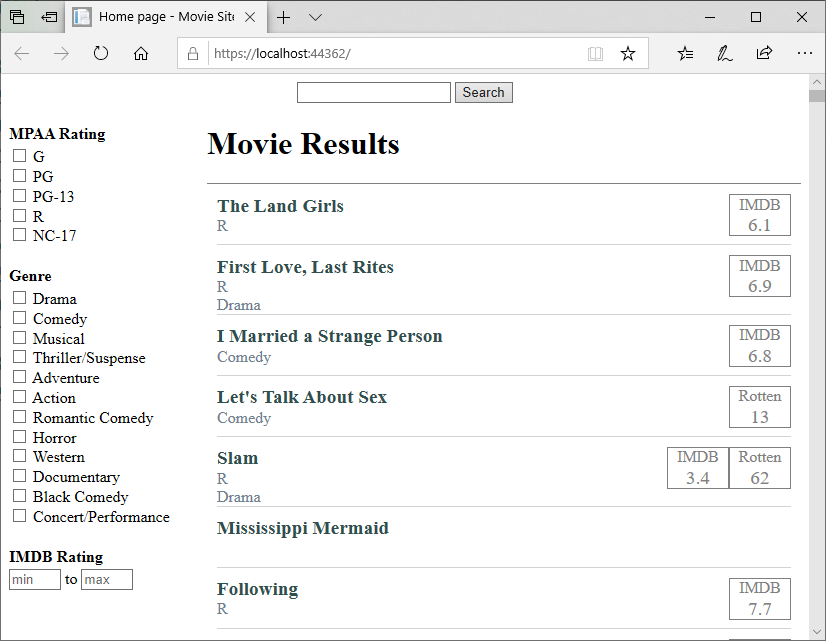
Capturing the Filter Values
Now we need to get the filter values from our GET request query string. We could do this like we’ve been doing before, with:
Request.Query["IMDBMin"];
But the returned value would be a string, so we’d need to parse it:
IMDBMin = double.Parse(Request.Query["IMDBMin"]);
If the query was null
, then this would evaluate to NaN
, which we wouldn’t want to set our <input>
to…
Instead, we’ll look at some options built into the PageModel.
Parameter Binding
The first of these options is Parameter Binding. In this approach, we define parameters to our OnGet()
method to be parsed out of the request automatically, i.e.:
/// <summary>
/// Gets the search results for display on the page
/// </summary>
public void OnGet(string SearchTerms, string[] MPAARatings, string[] Genres, double? IMDBMin, double? IMDBMax) {
this.SearchTerms = SearchTerms;
this.MPAARatings = MPAARatings;
this.Genres = Genres;
this.IMDBMin = IMDBMin;
this.IMDBMax = IMDBMax;
Movies = MovieDatabase.Search(SearchTerms);
Movies = MovieDatabase.FilterByMPAARating(Movies, MPAARatings);
Movies = MovieDatabase.FilterByGenre(Movies, Genres);
Movies = MovieDatabase.FilterByIMDBRating(Movies, IMDBMin, IMDBMax);
}
The benefit of this approach is that as long as C# knows a conversion into the type we specify, the conversion is done automatically. Note that the parameter name matches the name
property of the corresponding <input>
- this must be the case for the Razor Page to bind the parameter to the corresponding input value.
Note that we still need to assign these parameter values to the corresponding properties of our PageModel. If we don’t, then those properties will all be null
, and the <inputs>
rendered on our page will always be blank.
Model Binding
A second option is to use Model Binding. Model binding also automatically converts incoming form data, but in this case it binds directly to the properties of our PageModel. We indicate this form of binding with a [BindProperty]
attribute, i.e.:
public class IndexModel : PageModel {
[BindProperty(SupportsGet=true)]
public string SearchTerms {get; set;}
[BindProperty(SupportsGet=true)]
public string[] MPAARatings {get; set;}
[BindProperty(SupportsGet=true)]
public string[] Genres {get; set;}
[BindProperty(SupportsGet=true)]
public double? IMDBMin {get; set;}
[BindProperty(SupportsGet=true)]
public double? IMDBMax {get; set;}
/// <summary>
/// Gets the search results for display on the page
/// </summary>
public void OnGet() {
Movies = MovieDatabase.Search(SearchTerms);
Movies = MovieDatabase.FilterByMPAARating(Movies, MPAARatings);
Movies = MovieDatabase.FilterByGenre(Movies, Genres);
Movies = MovieDatabase.FilterByIMDBRating(Movies, IMDBMin, IMDBMax);
}
}
Note that with this approach, the incoming data is directly bound to the properties, so we don’t need to do any special assignments within our OnGet()
method. Also, note that we have to use SupportsGet=true
in order for this binding to occur on GET requests (by default, model binding only happens with POST requests).
Note
You only need to do one binding approach per property in a PageModel. I.e. you can just use the property decorator:
public class SomePageModel : PageModel
{
[BindProperty(SupportsGet=true)]
public float SomeProperty { get; set; }
public void OnGet() {
DoSomething(SomeProperty);
}
}
or you might use parameter binding:
public class SomePageModel : PageModel
{
public void OnGet(float SomeProperty) {
DoSomething(SomeProperty);
}
}
or you can parse it from the request:
public class SomePageModel : PageModel
{
public void OnGet() {
var someProperty = float.Parse(Request.Query["SomeProperty"]);
DoSomething(someProperty);
}
}
These are all different means of accessing the same data from the incoming request.
Now all we need to do is implement the actual filter.
Implementing the IMDB Rating Filter
We’ll define the new filter in our MovieDatabase
class as another static method:
/// <summary>
/// Filters the provided collection of movies
/// to those with IMDB ratings falling within
/// the specified range
/// </summary>
/// <param name="movies">The collection of movies to filter</param>
/// <param name="min">The minimum range value</param>
/// <param name="max">The maximum range value</param>
/// <returns>The filtered movie collection</returns>
public static IEnumerable<Movie> FilterByIMDBRating(IEnumerable<Movie> movies, double? min, double? max)
{
// TODO: Filter movies
}
Notice that here too we use the nullable double value. So our first step is probably to do a null
check:
if (min == null && max == null) return movies;
But what if only one is null? Should we filter for that part of the range? It wouldn’t be hard to do:
var results = new List<Movie>();
// only a maximum specified
if(min == null)
{
foreach(Movie movie in movies)
{
if (movie.IMDBRating <= max) results.Add(movie);
}
return results;
}
And the minimum would mirror that:
// only a minimum specified
if(max == null)
{
foreach(Movie movie in movies)
{
if (movie.IMDBRating >= min) results.Add(movie);
}
return results;
}
Finally, we could handle the case where we have both a min and max value to our range:
// Both minimum and maximum specified
foreach(Movie movie in movies)
{
if(movie.IMDBRating >= min && movie.IMDBRating <= max)
{
results.Add(movie);
}
}
return results;
Notice too, that in each of these cases we’re treating the range as inclusive (including the specified minimum and maximum). This is the behavior most casual internet users will expect. If the database and user expectations are different for your audience, you’d want your code to match that expectation.
Now we can filter by IMDB rating:
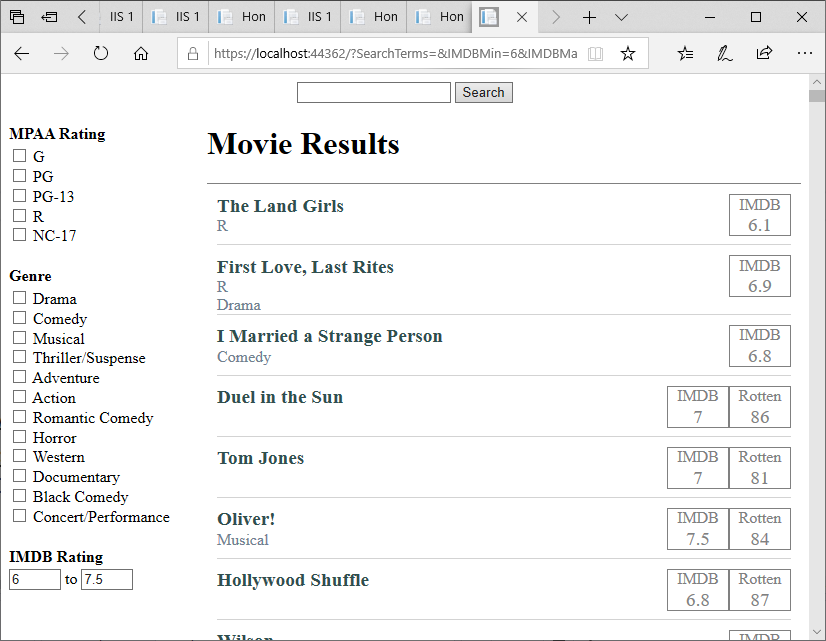
Finishing Up
Since we’re displaying the Rotten Tomatoes rating, we should probably also have a filter for it. This will work almost exactly like the IMDB rating - but with the range from 0 to 100. I’ll leave this as an exercise for the reader.
Numerical Filters
Let’s tackle one of the critics ratings next. While we could create categories and use checkboxes, this doesn’t capture the incremental values (i.e. 4.3), and it would be a lot of checkboxes for Rotten Tomatoes ratings! Instead, we’ll use a numerical filter, which limits our possible results to a range - between a minimum and maximum value.
Moreover, let’s clean up our Index page, as it is getting difficult to determine what filter(s) go together, and and are adding more.
Refactoring the Index Page
Let’s move the filters to a column on the left, leave the search bar above, and show our results on the right. This will require refactoring our Index.cshtml file:
<form id="movie-database">
<div id="search">
<input type="text" name="SearchTerms" value="@Model.SearchTerms" />
<input type="submit" value="Search">
</div>
<div id="filters">
<h4>MPAA Rating</h4>
@foreach (string rating in MovieDatabase.MPAARatings)
{
<label>
<input type="checkbox" name="MPAARatings" value="@rating" checked="@Model.MPAARatings.Contains(rating)" />
@rating
</label>
}
<h4>Genre</h4>
@foreach (string genre in MovieDatabase.Genres)
{
<label>
<input type="checkbox" name="Genres" value="@genre" checked="@Model.Genres.Contains(genre)" />
@genre
</label>
}
<h4>IMDB Rating</h4>
<div>
<input name="IMDBMin" type="number" min="0" max="10" step="0.1" placeholder="min"/>
to
<input name="IMDBMax" type="number" min="0" max="10" step="0.1" placeholder="max"/>
</div>
</div>
<div id="results">
<h1>Movie Results</h1>
<ul class="movie-list">
@foreach (Movie movie in @Model.Movies)
{
<li>
<div class="details">
<h3 class="title">@movie.Title</h3>
<div class="mpaa">@movie.MPAARating</div>
<div class="genre">@movie.MajorGenre</div>
</div>
<div class="ratings">
@if (movie.IMDBRating != null)
{
<div class="imdb">
@movie.IMDBRating
</div>
}
@if (movie.RottenTomatoesRating != null)
{
<div class="rotten-tomatoes">
@movie.RottenTomatoesRating
</div>
}
</div>
</li>
}
</ul>
</div>
</form>
Most of this is simply moving elements around the page, but note that we are using inputs of type=number
to represent our range of IMDB values. We can specify a minimum and maximum for this range, as well as an allowable increment. Also, we use the placeholder
attribute to put text into the input
until a value is added.
Adding More Styles
Now we’ll need to add some rules to our wwwroot/css/styles.css. First, we’ll use a grid
for the layout of the form:
form#movie-database {
display: grid;
grid-template-columns: 1fr 3fr;
grid-template-rows: auto auto;
}
The right column will be three times as big as the left.
We can make our search bar span both columns with grid-column-start
and grid-column-end
:
#search {
grid-column-start: 1;
grid-column-end: 3;
text-align: center;
}
Notice too that for CSS, we start counting at 1, not 0. The filters and the results will fall in the next row automatically, each taking up their own respective grid cell. You can read more about the grid layout in A Complete Guide to Grid.
Let’s go ahead and use flexbox to lay out our filters in a column:
#filters {
display: flex;
flex-direction: column;
}
And make our number inputs bigger:
#filters input[type=number] {
width: 4rem;
}
Notice the use of square brackets in our CSS Selector to only apply to inputs with type number.
Also, let’s add a margin above and remove most of the margin below our <h4>
elements:
#filters h4 {
margin-bottom: 0.2rem;
margin-top: 2rem;
}
The resulting page looks much cleaner:
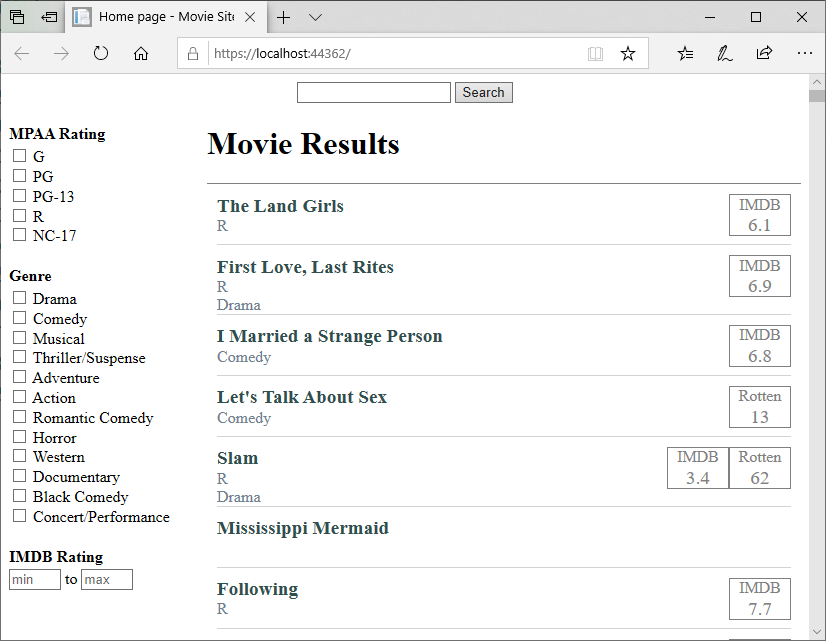
Capturing the Filter Values
Now we need to get the filter values from our GET request query string. We could do this like we’ve been doing before, with:
Request.Query["IMDBMin"];
But the returned value would be a string, so we’d need to parse it:
IMDBMin = double.Parse(Request.Query["IMDBMin"]);
If the query was null
, then this would evaluate to NaN
, which we wouldn’t want to set our <input>
to…
Instead, we’ll look at some options built into the PageModel.
Parameter Binding
The first of these options is Parameter Binding. In this approach, we define parameters to our OnGet()
method to be parsed out of the request automatically, i.e.:
/// <summary>
/// Gets the search results for display on the page
/// </summary>
public void OnGet(string SearchTerms, string[] MPAARatings, string[] Genres, double? IMDBMin, double? IMDBMax) {
this.SearchTerms = SearchTerms;
this.MPAARatings = MPAARatings;
this.Genres = Genres;
this.IMDBMin = IMDBMin;
this.IMDBMax = IMDBMax;
Movies = MovieDatabase.Search(SearchTerms);
Movies = MovieDatabase.FilterByMPAARating(Movies, MPAARatings);
Movies = MovieDatabase.FilterByGenre(Movies, Genres);
Movies = MovieDatabase.FilterByIMDBRating(Movies, IMDBMin, IMDBMax);
}
The benefit of this approach is that as long as C# knows a conversion into the type we specify, the conversion is done automatically. Note that the parameter name matches the name
property of the corresponding <input>
- this must be the case for the Razor Page to bind the parameter to the corresponding input value.
Note that we still need to assign these parameter values to the corresponding properties of our PageModel. If we don’t, then those properties will all be null
, and the <inputs>
rendered on our page will always be blank.
Model Binding
A second option is to use Model Binding. Model binding also automatically converts incoming form data, but in this case it binds directly to the properties of our PageModel. We indicate this form of binding with a [BindProperty]
attribute, i.e.:
public class IndexModel : PageModel {
[BindProperty(SupportsGet=true)]
public string SearchTerms {get; set;}
[BindProperty(SupportsGet=true)]
public string[] MPAARatings {get; set;}
[BindProperty(SupportsGet=true)]
public string[] Genres {get; set;}
[BindProperty(SupportsGet=true)]
public double? IMDBMin {get; set;}
[BindProperty(SupportsGet=true)]
public double? IMDBMax {get; set;}
/// <summary>
/// Gets the search results for display on the page
/// </summary>
public void OnGet() {
Movies = MovieDatabase.Search(SearchTerms);
Movies = MovieDatabase.FilterByMPAARating(Movies, MPAARatings);
Movies = MovieDatabase.FilterByGenre(Movies, Genres);
Movies = MovieDatabase.FilterByIMDBRating(Movies, IMDBMin, IMDBMax);
}
}
Note that with this approach, the incoming data is directly bound to the properties, so we don’t need to do any special assignments within our OnGet()
method. Also, note that we have to use SupportsGet=true
in order for this binding to occur on GET requests (by default, model binding only happens with POST requests).
Note
You only need to do one binding approach per property in a PageModel. I.e. you can just use the property decorator:
public class SomePageModel : PageModel
{
[BindProperty(SupportsGet=true)]
public float SomeProperty { get; set; }
public void OnGet() {
DoSomething(SomeProperty);
}
}
or you might use parameter binding:
public class SomePageModel : PageModel
{
public void OnGet(float SomeProperty) {
DoSomething(SomeProperty);
}
}
or you can parse it from the request:
public class SomePageModel : PageModel
{
public void OnGet() {
var someProperty = float.Parse(Request.Query["SomeProperty"]);
DoSomething(someProperty);
}
}
These are all different means of accessing the same data from the incoming request.
Now all we need to do is implement the actual filter.
Implementing the IMDB Rating Filter
We’ll define the new filter in our MovieDatabase
class as another static method:
/// <summary>
/// Filters the provided collection of movies
/// to those with IMDB ratings falling within
/// the specified range
/// </summary>
/// <param name="movies">The collection of movies to filter</param>
/// <param name="min">The minimum range value</param>
/// <param name="max">The maximum range value</param>
/// <returns>The filtered movie collection</returns>
public static IEnumerable<Movie> FilterByIMDBRating(IEnumerable<Movie> movies, double? min, double? max)
{
// TODO: Filter movies
}
Notice that here too we use the nullable double value. So our first step is probably to do a null
check:
if (min == null && max == null) return movies;
But what if only one is null? Should we filter for that part of the range? It wouldn’t be hard to do:
var results = new List<Movie>();
// only a maximum specified
if(min == null)
{
foreach(Movie movie in movies)
{
if (movie.IMDBRating <= max) results.Add(movie);
}
return results;
}
And the minimum would mirror that:
// only a minimum specified
if(max == null)
{
foreach(Movie movie in movies)
{
if (movie.IMDBRating >= min) results.Add(movie);
}
return results;
}
Finally, we could handle the case where we have both a min and max value to our range:
// Both minimum and maximum specified
foreach(Movie movie in movies)
{
if(movie.IMDBRating >= min && movie.IMDBRating <= max)
{
results.Add(movie);
}
}
return results;
Notice too, that in each of these cases we’re treating the range as inclusive (including the specified minimum and maximum). This is the behavior most casual internet users will expect. If the database and user expectations are different for your audience, you’d want your code to match that expectation.
Now we can filter by IMDB rating:
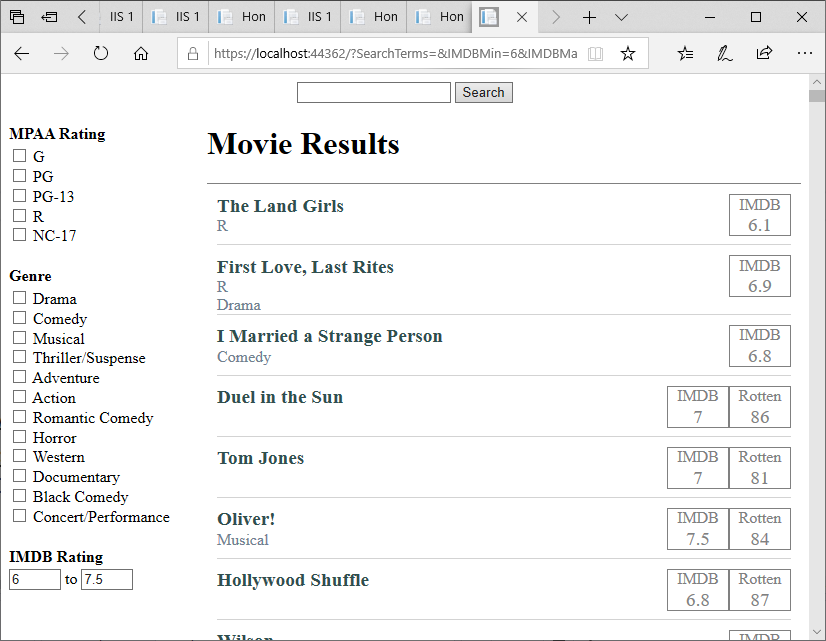
Finishing Up
Since we’re displaying the Rotten Tomatoes rating, we should probably also have a filter for it. This will work almost exactly like the IMDB rating - but with the range from 0 to 100. I’ll leave this as an exercise for the reader.
Numerical Filters
Let’s tackle one of the critics ratings next. While we could create categories and use checkboxes, this doesn’t capture the incremental values (i.e. 4.3), and it would be a lot of checkboxes for Rotten Tomatoes ratings! Instead, we’ll use a numerical filter, which limits our possible results to a range - between a minimum and maximum value.
Moreover, let’s clean up our Index page, as it is getting difficult to determine what filter(s) go together, and are adding more.
Refactoring the Index Page
Let’s move the filters to a column on the left, leave the search bar above, and show our results on the right. This will require refactoring our Index.cshtml file:
<form id="movie-database">
<div id="search">
<input type="text" name="SearchTerms" value="@Model.SearchTerms" />
<input type="submit" value="Search">
</div>
<div id="filters">
<h4>MPAA Rating</h4>
@foreach (string rating in MovieDatabase.MPAARatings)
{
<label>
<input type="checkbox" name="MPAARatings" value="@rating" checked="@Model.MPAARatings.Contains(rating)" />
@rating
</label>
}
<h4>Genre</h4>
@foreach (string genre in MovieDatabase.Genres)
{
<label>
<input type="checkbox" name="Genres" value="@genre" checked="@Model.Genres.Contains(genre)" />
@genre
</label>
}
<h4>IMDB Rating</h4>
<div>
<input name="IMDBMin" type="number" min="0" max="10" step="0.1" placeholder="min"/>
to
<input name="IMDBMax" type="number" min="0" max="10" step="0.1" placeholder="max"/>
</div>
</div>
<div id="results">
<h1>Movie Results</h1>
<ul class="movie-list">
@foreach (Movie movie in @Model.Movies)
{
<li>
<div class="details">
<h3 class="title">@movie.Title</h3>
<div class="mpaa">@movie.MPAARating</div>
<div class="genre">@movie.MajorGenre</div>
</div>
<div class="ratings">
@if (movie.IMDBRating != null)
{
<div class="imdb">
@movie.IMDBRating
</div>
}
@if (movie.RottenTomatoesRating != null)
{
<div class="rotten-tomatoes">
@movie.RottenTomatoesRating
</div>
}
</div>
</li>
}
</ul>
</div>
</form>
Most of this is simply moving elements around the page, but note that we are using inputs of type=number
to represent our range of IMDB values. We can specify a minimum and maximum for this range, as well as an allowable increment. Also, we use the placeholder
attribute to put text into the input
until a value is added.
Adding More Styles
Now we’ll need to add some rules to our wwwroot/css/site.css. First, we’ll use a grid
for the layout of the form:
form#movie-database {
display: grid;
grid-template-columns: 1fr 3fr;
grid-template-rows: auto auto;
}
The right column will be three times as big as the left.
We can make our search bar span both columns with grid-column-start
and grid-column-end
:
#search {
grid-column-start: 1;
grid-column-end: 3;
text-align: center;
}
Notice too that for CSS, we start counting at 1, not 0. The filters and the results will fall in the next row automatically, each taking up their own respective grid cell. You can read more about the grid layout in A Complete Guide to Grid.
Let’s go ahead and use flexbox to lay out our filters in a column:
#filters {
display: flex;
flex-direction: column;
}
And make our number inputs bigger:
#filters input[type=number] {
width: 4rem;
}
Notice the use of square brackets in our CSS Selector to only apply to inputs with type number.
Also, let’s add a margin above and remove most of the margin below our <h4>
elements:
#filters h4 {
margin-bottom: 0.2rem;
margin-top: 2rem;
}
The resulting page looks much cleaner:
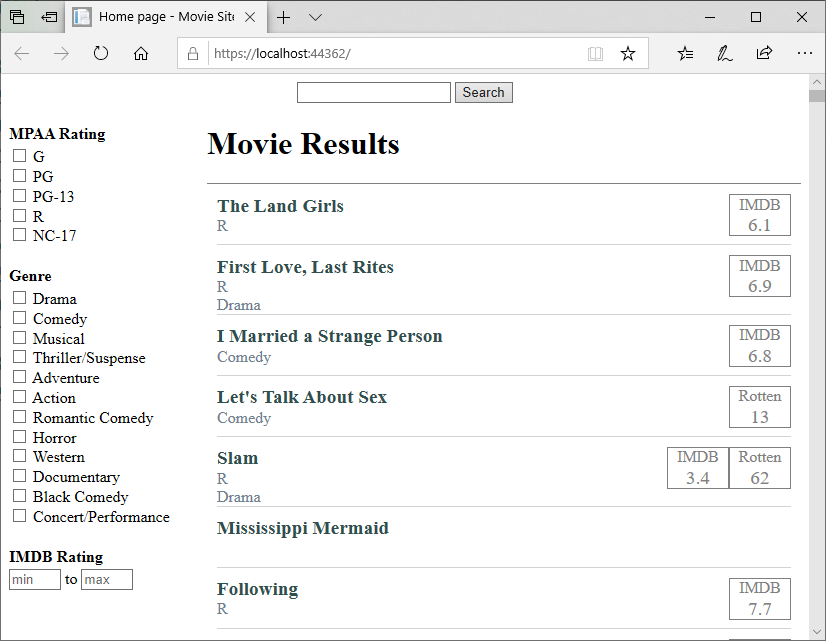
Capturing the Filter Values
Now we need to get the filter values from our GET request query string. We could do this like we’ve been doing before, with:
Request.Query["IMDBMin"];
But the returned value would be a string, so we’d need to parse it:
IMDBMin = double.Parse(Request.Query["IMDBMin"]);
If the query was null
, then this would evaluate to NaN
, which we wouldn’t want to set our <input>
to…
Instead, we’ll look at some options built into the PageModel.
Parameter Binding
The first of these options is Parameter Binding. In this approach, we define parameters to our OnGet()
method to be parsed out of the request automatically, i.e.:
/// <summary>
/// Gets the search results for display on the page
/// </summary>
public void OnGet(string SearchTerms, string[] MPAARatings, string[] Genres, double? IMDBMin, double? IMDBMax) {
this.SearchTerms = SearchTerms;
this.MPAARatings = MPAARatings;
this.Genres = Genres;
this.IMDBMin = IMDBMin;
this.IMDBMax = IMDBMax;
Movies = MovieDatabase.Search(SearchTerms);
Movies = MovieDatabase.FilterByMPAARating(Movies, MPAARatings);
Movies = MovieDatabase.FilterByGenre(Movies, Genres);
Movies = MovieDatabase.FilterByIMDBRating(Movies, IMDBMin, IMDBMax);
}
The benefit of this approach is that as long as C# knows a conversion into the type we specify, the conversion is done automatically. Note that the parameter name matches the name
property of the corresponding <input>
- this must be the case for the Razor Page to bind the parameter to the corresponding input value.
Note that we still need to assign these parameter values to the corresponding properties of our PageModel. If we don’t, then those properties will all be null
, and the <inputs>
rendered on our page will always be blank.
Model Binding
A second option is to use Model Binding. Model binding also automatically converts incoming form data, but in this case it binds directly to the properties of our PageModel. We indicate this form of binding with a [BindProperty]
attribute, i.e.:
public class IndexModel : PageModel {
[BindProperty(SupportsGet=true)]
public string SearchTerms {get; set;}
[BindProperty(SupportsGet=true)]
public string[] MPAARatings {get; set;}
[BindProperty(SupportsGet=true)]
public string[] Genres {get; set;}
[BindProperty(SupportsGet=true)]
public double? IMDBMin {get; set;}
[BindProperty(SupportsGet=true)]
public double? IMDBMax {get; set;}
/// <summary>
/// Gets the search results for display on the page
/// </summary>
public void OnGet() {
Movies = MovieDatabase.Search(SearchTerms);
Movies = MovieDatabase.FilterByMPAARating(Movies, MPAARatings);
Movies = MovieDatabase.FilterByGenre(Movies, Genres);
Movies = MovieDatabase.FilterByIMDBRating(Movies, IMDBMin, IMDBMax);
}
}
Note that with this approach, the incoming data is directly bound to the properties, so we don’t need to do any special assignments within our OnGet()
method. Also, note that we have to use SupportsGet=true
in order for this binding to occur on GET requests (by default, model binding only happens with POST requests).
Note
You only need to do one binding approach per property in a PageModel. I.e. you can just use the property decorator:
public class SomePageModel : PageModel
{
[BindProperty(SupportsGet=true)]
public float SomeProperty { get; set; }
public void OnGet() {
DoSomething(SomeProperty);
}
}
or you might use parameter binding:
public class SomePageModel : PageModel
{
public void OnGet(float SomeProperty) {
DoSomething(SomeProperty);
}
}
or you can parse it from the request:
public class SomePageModel : PageModel
{
public void OnGet() {
var someProperty = float.Parse(Request.Query["SomeProperty"]);
DoSomething(someProperty);
}
}
These are all different means of accessing the same data from the incoming request.
Now all we need to do is implement the actual filter.
Implementing the IMDB Rating Filter
We’ll define the new filter in our MovieDatabase
class as another static method:
/// <summary>
/// Filters the provided collection of movies
/// to those with IMDB ratings falling within
/// the specified range
/// </summary>
/// <param name="movies">The collection of movies to filter</param>
/// <param name="min">The minimum range value</param>
/// <param name="max">The maximum range value</param>
/// <returns>The filtered movie collection</returns>
public static IEnumerable<Movie> FilterByIMDBRating(IEnumerable<Movie> movies, double? min, double? max)
{
// TODO: Filter movies
}
Notice that here too we use the nullable double value. So our first step is probably to do a null
check:
if (min == null && max == null) return movies;
But what if only one is null? Should we filter for that part of the range? It wouldn’t be hard to do:
var results = new List<Movie>();
// only a maximum specified
if(min == null)
{
foreach(Movie movie in movies)
{
if (movie.IMDBRating <= max) results.Add(movie);
}
return results;
}
And the minimum would mirror that:
// only a minimum specified
if(max == null)
{
foreach(Movie movie in movies)
{
if (movie.IMDBRating >= min) results.Add(movie);
}
return results;
}
Finally, we could handle the case where we have both a min and max value to our range:
// Both minimum and maximum specified
foreach(Movie movie in movies)
{
if(movie.IMDBRating >= min && movie.IMDBRating <= max)
{
results.Add(movie);
}
}
return results;
Notice too, that in each of these cases we’re treating the range as inclusive (including the specified minimum and maximum). This is the behavior most casual internet users will expect. If the database and user expectations are different for your audience, you’d want your code to match that expectation.
Now we can filter by IMDB rating:
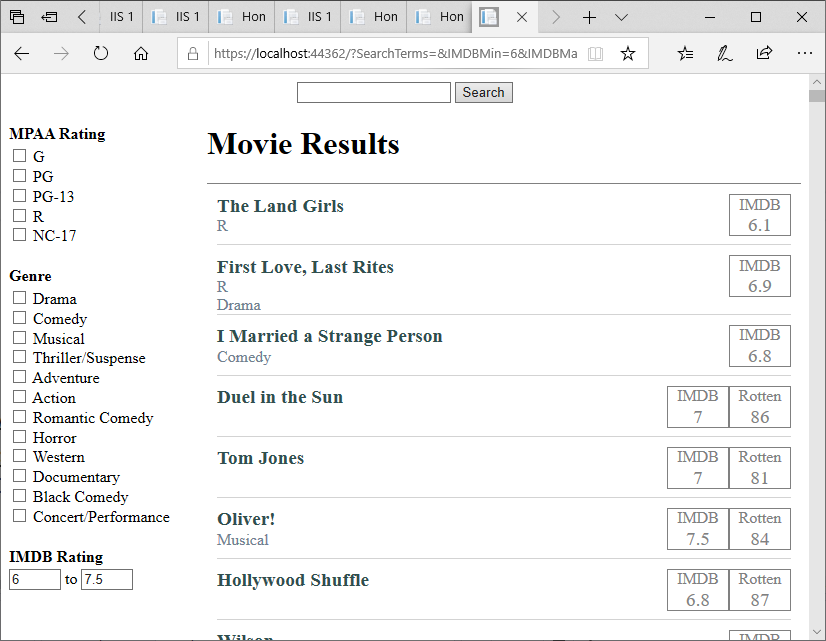
Finishing Up
Since we’re displaying the Rotten Tomatoes rating, we should probably also have a filter for it. This will work almost exactly like the IMDB rating - but with the range from 0 to 100. I’ll leave this as an exercise for the reader.
Using LINQ
In our movie website, we’ve been using a custom “database” object, MovieDatabase
to provide the movie data and search and filter functionality. In professional practice, we would probably replace this with an external database program. These programs have been developed specifically for persisting and accessing data, and leverage specialized data structures like B+ trees that allow them to do searches and filters much more efficiently.
Some of the most widely adopted database programs are relational databases like MySQL, MsSQL, Postgres, and SQLLite. These predate object-orientation, and accept requests for data in the form of SQL (Structured Query Language). You will learn more about these databases and how to use them in CIS560.
LINQ
Microsoft, inspired by SQL, introduced Language Integrated Query (LINQ) in 2007 to bring the concept of a query language into .NET. LINQ allows you to construct queries in either chained method calls or in a syntax similar to SQL that can operate on collections or relational databases.
For relational databases, LINQ queries are converted internally to SQL queries, and dispatched to the database. But their real power is that they can also be used with any collection that supports either the IEnumerable
or IEnumerable<T>
interfaces. LINQ can be used to replace the kinds of filter and search functions we wrote for our MovieDatabase
. Let’s try it out with our movie website.
LINQ operates through extension methods, so we need to be sure to add the LINQ namespace to our CS files where we are going to employ them. We’ll be adding our LINQ commands to our page model, so sure that is the case with your Pages/Index.cshtml.cs:
Adding this using statement will bring in all the extension methods for LINQ, and will also prompt Visual Studio to recognize the query-style syntax.
Searching with LINQ
One of the most useful extension methods in LINQ is Where(). It can be invoked on any IEnumerable
and accepts a Func
(a delegate type). This delegate takes a single argument - the current item being enumerated over, and its body should return a boolean value - true
if the item should be included in the results, and false
if it should not.
We can thus use our existing SearchTerms
property as the basis for our test: movie => movie.Title != null && movie.Title.Contains(SearchTerms)
(note that with lambda expressions, if the result is single expression we can omit the {}
and the return
is implied). Also note that we incorporate the null check directly into the expression using the boolean and &&
operator.
We can replace our current search with a Where()
call invoking this method:
Movies = MovieDatabase.All.Where(movie => movie.Title != null && movie.Title.Contains(SearchTerms, String));
However if we run the program we will encounter a null exception. On our first GET request, the value of SearchTerms
is null, which is not a legal argument for String.Contains()
.
Making Search Conditional
If the SearchTerms
is null, that indicates that the user did not enter a search term - accordingly, we probably don’t want to search. We can wrap our filter in an if
test to prevent applying the filter when there is no search term. Thus, we would refactor our filtering expression to:
Movies = MovieDatabase.All;
// Search movie titles for the SearchTerms
if(SearchTerms != null) {
Movies = Movies.Where(movie => movie.Title != null && movie.Title.Contains(SearchTerms, StringComparison.InvariantCultureIgnoreCase));
}
Now if we run the program, and search for a specific term, we’ll see our results is modified to contain only those movies with the terms in their title!
We can also use the query syntax instead of the extension method syntax, which is similar to SQL:
Movies = MovieDatabase.All;
// Search movie titles for the SearchTerms
if(SerchTerms != null)
{
Movies = from movie in Movies
where movie.Title != null && movie.Title.Contains(SearchTerms, StringComparison.InvariantCultureIgnoreCase)
select movie;
}
This syntax is converted by the C# compiler to use the extension methods as part of the compilation process.
Info
The search approach listed above will only find the exact search terms, i.e. searching for “Clear Present Danger” will not match the film “Clear and Present Danger”. How might you tweak the search approach so that terms would be found individually?
You can use either form for writing your queries, though it is best to stay consistent within a single program.
Filtering with LINQ
Filtering is also accomplished through the Where(). We can add additional tests within our search Where()
, but it is more legible to add additional Where
calls:
Movies = MovieDatabase.All
// Search movie titles for the SearchTerms
if(SearchTerms != null) {
Movies = Movies.Where(movie => movie.Title != null && movie.Title.Contains(SearchTerms, StringComparison.InvariantCultureIgnoreCase));
}
// Filter by MPAA Rating
if(MPAARatings != null && MPAARatings.Length != 0)
{
Movies = Movies.Where(movie =>
movie.MPAARating != null &&
MPAARatings.Contains(movie.MPAARating)
);
}
The numerical filters are handled the same way - with additional Where
clauses. I leave this as an exercise for the reader to complete.
Benefits of LINQ
While LINQ is a bit less code for us to write, there is another big benefit - the actual filtering is only applied when we start iterating through the results. This means that LINQ queries with multiple Where()
invocations can combine them into a single iteration.
Consider our movie website example. We have four filters - the search, the MPAA Ratings, the IMDB Ratings, and the Rotten Tomato Rating. In the worst case, each movie in the database would pass each filter, so that our list never got smaller. With the filter functions we wrote in MovieDatabase
, we would have to iterate over that full list four times. In terms of complexity, that’s $O(4n)$.
In contrast, because LINQ doesn’t actually run the filters until we start iterating, it can combine all the While
tests into a single boolean expression. The result is it only has to iterate through the list once. Hence, its complexity becomes $O(n)$. There is a little additional overhead for holding onto the query information that way, but it’s small compared to the benefit.
Also, we could have done the same kind of optimization ourselves, but it takes a lot of work to set up, and may not be worth it for a single web app. But LINQ provides us that benefit for free.